Multidisciplinary approach to vascular rings and vascular-related aerodigestive compression: a clinical practice review
Introduction
Vascular rings and vascular-related aerodigestive compression syndromes encompass a variety of lesions that involve abnormal development of the aortic arch with resultant vascular compression of either the trachea, the esophagus, or both. True, or complete, vascular rings include double aortic arch and right aortic arch with aberrant left subclavian artery and left ligamentum arteriosum and have a prevalence of approximately 1–1.3 per 10,000 live births (1,2). Beyond vascular rings, aerodigestive compression syndromes include congenital lesions as well as acquired lesions. In many of these other aerodigestive compression syndromes, the true incidence of the underlying pathology is unknown given the potential for underdiagnosis and the broad spectrum of symptom severity.
Despite the substantial variability in etiologies and presentations, a multidisciplinary approach to diagnostic evaluation has been standardized at Boston Children’s Hospital. Herein, we review the clinical presentation and diagnostic evaluation followed by treatment options for vascular ring and aerodigestive compression syndromes.
Clinical presentation and diagnostic evaluation
Patients with a true vascular ring would typically present with symptoms of tracheal and/or esophageal compression. Patients with double aortic arch often present early in infancy with severe symptoms whereas patients with right aortic arch and aberrant left subclavian arteries may present symptoms later on. Moreover, patients with incomplete rings often present later in childhood or are asymptomatic and only incidentally diagnosed. As a whole, respiratory symptoms, e.g., noisy breathing, stridor, cough, or recurrent upper respiratory infections, are present in 88–94% of patients. Additionally, 43–50% of patients will have esophageal symptoms including dysphagia and choking (3-6). However, up to 47% to 66% of adult patients with aberrant right subclavian will present primarily with esophageal symptoms (6-8). A challenge that the clinician may face with respect to eliciting symptoms is that patients may have been labeled as “picky” or “slow eaters” or carry a diagnosis of reflux (4), and so the exact symptomatology may be underappreciated.
Echocardiography is a useful initial screening tool for identifying arch sidedness and presence of an aberrant subclavian artery. However, axial imaging—either computed tomographic angiography or magnetic resonance angiography—is necessary to delineate the vascular anatomy and the relationship with the airway and esophagus. Efforts have been made to use dynamic computed tomography to detect airway malacia, but rigid dynamic tracheobronchoscopy remains the gold-standard for airway assessment (9-12).
Tracheobronchoscopy is the technique of choice for the definitive diagnosis of tracheobronchomalacia and tracheobronchial compression. Our group performs a three-phase bronchoscopy under general anesthesia without muscle relaxation. In the first phase, the rigid ventilating bronchoscope is introduced with the patient breathing quietly. This reveals baseline areas of compression, abnormalities of cartilage shape, and presence of granulation tissue. In the second phase, coughing is induced by decreasing the depth of anesthesia in order to reveal the dynamic component of the airway collapse that is typical of tracheobronchomalacia. The final phase of the bronchoscopy is to distend the airway with positive pressure. This allows for identification of areas of fixed tracheobronchial compression and also presence of tracheoesophageal fistulae, tracheal diverticula, or abnormal branching patterns (13).
Our team uses a detailed standardized reporting process. The trachea is divided into 3 segments. Between the cricoid and the first rib is labeled T1; from the first rib to the level of the innominate artery is T2; and T3 is from the innominate artery down to the carina. The carina is described distinct from T3. The left mainstem bronchus is divided into thirds: L1, L2, and L3. While there are fewer anatomic landmarks for the left mainstem bronchus, L2 represents the region that typically crosses over a left-sided descending thoracic aorta. The right mainstem bronchus is divided in half: R1 and R2 (Figure 1) (14). All bronchoscopies are video-recorded. Percent compression of the airway for each of these regions is reported during each of the three phases of the evaluation. Given the subjective nature of the diagnosis of tracheobronchomalacia, a common terminology and reporting system is essential for communication between providers.
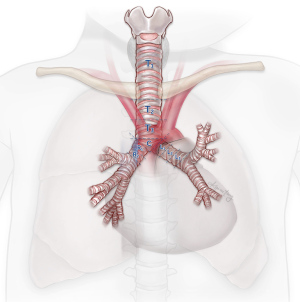
An esophagram may demonstrate the location of an obstruction in patients with esophageal symptoms. An impression on the esophagus by esophagram correlated with the presence of an aberrant subclavian artery by axial imaging is typically diagnostic (Figure 2). However, in select patients, liquids may pass easily despite the presence of symptoms. In these cases, we have found that an esophageal transit study with incorporation of Technetium-99m sulfur colloid mixed into a solid food bolus, e.g., eggs, may aid in the elaboration of impaired transit (Figure 3). Flexible endoscopy can help determine whether the obstruction is fixed or not with insufflation and may also reveal other potential causes for dysphagia, e.g., eosinophilic esophagitis.
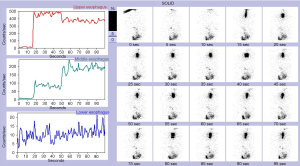
As part of the preoperative process, an otorhinolaryngologist will perform a vocal cord check and assess for laryngeal cleft. Vocal cord dysfunction may be congenital in nature, but more importantly patients who have had prior vascular ring or aortic arch operations may have preexisting vocal cord dysfunction that must be noted. If there is concern for aspiration, functional testing with a modified barium swallow is performed. We have found that standard pulmonary function tests generally are not helpful, as central airway collapse may not correlate with airflow obstruction in patients with known tracheobronchomalacia (15-17), and may not be consistent among younger patients (18-20). The utility of exercise pulmonary function tests is currently being evaluated.
Vascular rings
Double aortic arch
Double aortic arch represents between 31% and 58% of complete vascular rings (3,21-23). The two arches pass to either side of the trachea and esophagus causing compression (Figure 4). The right aortic arch is dominant in 71% to 89% of patients, and between 1% and 18% of patients are codominant. Through a muscle-sparing thoracotomy on the side of the non-dominant arch, the posterior aspect of the non-dominant arch is circumferentially mobilized. Prior to ligation and division, the diminutive arch may be test clamped in order to assess the adequacy of the remaining arch by measuring upper and lower extremity blood pressures. A short segment of the non-dominant aortic arch may be resected flush with both the subclavian artery and the descending thoracic aorta in order to avoid the development of compressive symptoms related to aneurysmal dilation of a residual stump, and each side is oversewn. A left ligamentum arteriosum is typically ligated and divided if the left arch is divided. In a patient with a left dominant aortic arch, the non-dominant right aortic arch is approached through a right thoracotomy, we do not routinely attempt to ligate and divide a left ligamentum arteriosum as division of the right arch completely relieves the ring. An additional concern, discussed below, relates to the position of the descending thoracic aorta.
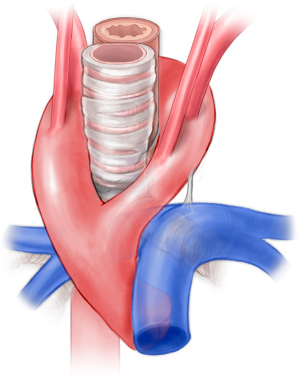
Right aortic arch aberrant left subclavian artery
Right aortic arch occurs in approximately 0.1% of the general population but may occur in up to 34–36% of patients with congenital heart disease, most prominently truncus arteriosus and tetralogy of Fallot (24-26). The proportion of patients with right aortic arch and aberrant left subclavian ranges from 14.1% to 66%. In the majority of these patients (50% to 90%), there is a left-sided ligamentum arteriosum forming a true vascular ring (Figure 5) (27-30). This arrangement constitutes between 51% and 63% of vascular ring cases (3,21-23). One approach has been to simply divide the ligamentum arteriosum (31). We do not recommend this approach as scar tissue may form in the area of the divided ligamentum, and ongoing compression may be present from the aberrant subclavian artery. We have noted this to be a frequent reason patients are referred to our facility with persistent symptoms following vascular ring repair.
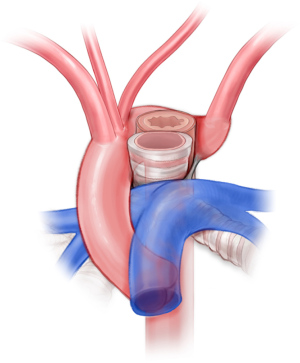
Patients with right aortic arch and aberrant left subclavian are approached via posterolateral left-sided muscle-sparing thoracotomy. After mobilizing the left subclavian artery, the ligamentum arteriosum is identified and divided. The esophagus is mobilized, and the fibrous bands causing constriction of the esophagus are divided. The base of the left subclavian is clamped aggressively in order to transect the vessel flush with the aorta. Otherwise, there is a risk of a residual stump and symptom recurrence (32,33). Subclavian-to-carotid transposition is performed. Depending on the position of the descending thoracic aorta, additional descending aortopexy may be indicated to distract the aorta away from the esophagus or airway (34,35).
Despite the initial enthusiasm for video assisted thoracoscopic approaches reported by Burke et al. from our institution (36), this approach is no longer routine (5). The ability to completely resect the diverticulum of Kommerell and also to perform vascular reconstruction is limited via a videoscopic approach. Moreover, adjunctive procedures such as aortopexy and tracheopexy may be challenging.
Notably, between 33% and 85% of patients with a right aortic arch will have mirror image branching (27-30). In the majority of these patients, a vascular ring does not exist. Rarely, there may be a ductus arteriosus connecting the left pulmonary artery to the descending thoracic aorta creating a vascular ring (37-40).
Aerodigestive compression syndromes, including incomplete vascular rings
Innominate artery compression syndrome and narrow sterno-cervical spine distance
The innominate artery passes anterior to the trachea. Among patients under 3 years of age, up to 44% of infants will have flattening of the airway by either lateral chest X-ray or computed tomography with an anteroposterior diameter that is less than the transverse diameter in 88% of patients (41,42). As patients age, the origin of the innominate artery translates rightward, and the anteroposterior diameter of the trachea is greater than or equal to the transverse diameter in 55% of patients older than 20 years. Despite the frequency of tracheal deformation, surgery is unnecessary in the vast majority of cases (43).
Innominate arteriopexy was first performed through a left anterolateral thoracotomy with reported complete resolution of the respiratory symptoms (44). Contemporary reports of innominate arteriopexy have reported complete response in approximately 62% and partial response in the remainder (45). In scenarios with significant tracheal compression, direct anterior tracheopexy in addition to aortopexy or innominate arteriopexy may be necessary (46). Innominate artery reimplantation on the distal ascending aorta is an alternative with reported complete response up to 86–93% (47-49).
A related phenomenon manifests in a subset of patients with chest wall or spine deformities with or without neuromuscular or neurological disorders, e.g., spastic cerebral palsy and straight back syndrome, in which the sternum to anterior cervical spine distance is reduced at the level of the thoracic inlet. Among these patients, either the sternum itself or the innominate artery may cause deformation of the trachea (50-54). This close relationship between the innominate artery and trachea is such that among patients with a sternum to anterior cervical spine distance of <2 cm, there appears to be an increased risk of tracheoinnominate fistula in patients who undergo a tracheostomy (55,56). Our group has proposed a metric that accounts for the patient’s body size and is termed the thoracic inlet index, similar to the Haller index (thoracic inlet index = width of first ribs/narrowest anteroposterior measurement from the center of the manubrium to the spine).
Maneuvers to address this deformity may include splitting and distracting the manubrium (54), expansion sternoplasty, rib grafting, manubrial resection, or methylmethacrylate sternal expansion with or without innominate artery reimplantation (50), and—in patients with hyperlordosis or opisthotonus—posterior spinal fusion (53). The approach at Boston Children’s Hospital has included a combination of orthopedic surgery, plastic surgery, general surgery, and cardiac surgery to perform sternal expansion with either rib autograft or iliac crest homograft, innominate artery reimplantation, tracheopexy, and pectoralis advancement flap (Figure 6).
Dysphagia lusoria (left aortic arch with aberrant right subclavian artery)
In 1787, David Bayford reported on the case of a woman who experienced severe dysphagia. At autopsy, he identified a left aortic arch and aberrant right subclavian causing “obstruction in deglutition” (57). He termed this dysphagia lusoria. Though this entity is typically associated with solid food dysphagia, we have noticed in young children that dysphagia with liquids and associated coughing or choking may be a common presentation. The left aortic arch and aberrant right subclavian artery may be termed an incomplete vascular ring as a right-sided ligamentum arteriosum, forming a true ring, is rare (58-61). Despite knowledge of this anatomical obstruction, the first repair of aberrant right subclavian artery was not performed until 1945 (62). During this operation, the subclavian artery was ligated proximal to the vertebral artery without reimplantation. Ligation and division of the subclavian artery without reimplantation is generally not recommended given the potential for subclavian steal syndrome, ischemic symptoms, or limb length discrepancy (63-67).
The approach to address the aberrant right subclavian artery mirrors the approach to aberrant left subclavian artery. Through a right posterolateral muscle-sparing thoracotomy, the aberrant subclavian artery and the esophagus are mobilized. The thoracic duct will frequently travel close to the takeoff of the aberrant subclavian artery, and great care must be taken to avoid injuring this structure. Subclavian-to-carotid transposition is performed, and intraoperative bronchoscopy is performed to determine whether aortopexy or tracheopexy is necessary.
In the adult literature, the hybrid approach is increasingly being employed to address symptomatic aberrant subclavian artery with or without a diverticulum of Kommerell. With the hybrid approach, a carotid-to-subclavian bypass is performed, and the subclavian artery proximal to the vertebral artery is then ligated. The diverticulum is then excluded with a thoracic endograft. Outcomes with the hybrid approach have been reported to be similar to open surgical repair with respect to both symptomatic relief and periprocedural mortality (8,68,69). An important consideration remains the increased late hazard for mortality and reintervention with thoracic endovascular aortic repair compared with open surgical repair for patients with descending thoracic aortic pathology (70). As such, while a hybrid approach may offer advantages over open surgery in older patients, hybrid approaches are best restricted to children who are deemed poor surgical candidates (71,72).
Midline descending aorta, hairpin aorta, and circumflex aorta
The aorta normally passes leftward of the trachea and continues as the descending thoracic aorta left of the spine. When the descending thoracic aorta is midline and directly anterior to the spine, there may be posterior intrusion into the carina or left mainstem bronchus and also compression of the esophagus (73,74). In patients with a hairpin aorta, the ascending and descending thoracic aorta are in close proximity forming a hairpin and causing the intervening structures to be compressed, this is often in the setting of a right arch with right descending aorta resulting in right mainstem bronchial compression but may also occur with left arch and left descending aorta resulting in left mainstem bronchial compression (75-77). When the descending thoracic aorta is on the opposite side of the aortic arch with a retroesophageal aortic segment, it is termed a circumflex aorta (28). Notably, this phenomenon may also arise in the setting of double aortic arch with a dominant right arch but a left-sided descending thoracic aorta. Division of the non-dominant left arch creates a circumflex aorta arrangement with the potential for ongoing compression of the trachea and esophagus despite relief of the vascular ring.
This class of aortic arch anomalies requires a thorough review of axial imaging. At Boston Children’s Hospital, the use of 3-dimensional segmentation software (Mimics, Materialise NV, Leuven, Belgium) is a critical part of the operative planning process (Figure 7). Additionally, given the potential risk for paraplegia with mobilization of the descending thoracic aorta, computed tomographic angiography is routinely used to identify the artery of Adamkiewicz, as discussed below.
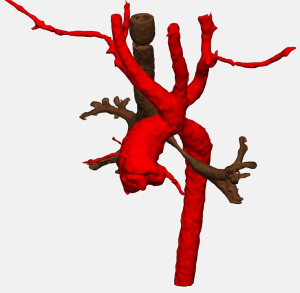
For patients with midline descending thoracic aorta or hairpin aorta with tracheobronchial compression, descending aortic translocation may be performed (74,78,79). Following median sternotomy, the aortic arch is cannulated along with a single right atrial venous cannula; the patient is then cooled to 28 degrees. The distal arch is transected between clamps beyond the subclavian artery, and the proximal end is oversewn while the head and heart are perfused by the aortic cannula. The distal end is then passed underneath the carina and beneath the right pulmonary artery. The superior-most intercostal arteries may need to be ligated and divided to achieve this degree of mobility. A distal perfusion cannula may be inserted into the descending aorta to provide temporary perfusion to the lower body. The heart is then arrested with antegrade cardioplegia, and a transverse aortotomy is made above the sinotubular junction. Through the aortotomy, a longitudinal incision is made in the posterior ascending aorta paying close attention to the position of the left main coronary artery. An end-to-side anastomosis is then constructed between the descending thoracic aorta and the posterior ascending aorta. One risk of relocating the descending thoracic aorta under the right pulmonary artery is right pulmonary artery compression and stenosis. This has happened in one case at Boston Children’s Hospital, and the pulmonary artery was subsequently stented with complete resolution. Due to concerns regarding airway compression, a balloon was provisionally expanded in the right pulmonary artery while bronchoscopy was performed, a technique that has been described by O’Byrne et al. (80).
The aortic uncrossing procedure for circumflex aorta was first reported by Planché in 1984 (81,82), and since then several case series and case reports have been published (40,75,83-89). The approach is similar to that of the descending aortic translocation. However, due to the site of aortic reconstruction, we routinely cannulate the innominate artery for use of selective antegrade cerebral perfusion during the reconstruction rather than employing deep hypothermic circulatory arrest (84), which has been favored by others (75,83). The primary difference between the two operations is that the aorta is brought to the opposite side of the trachea rather than under it. For a patient with right aortic arch and left descending aorta, this results in a left aortic arch. After performing an aortotomy at the level of the distal ascending, the backwall of the aorta is reconstructed. The front wall often requires reconstruction with patch material, which may consist of thick patch pulmonary homograft, bovine pericardium, Dacron, or autologous pericardium.
Other efforts to address circumflex aorta have included ascending-to-descending bypass with ligation of the distal arch (77,90-93), aggressive aortopexy (94), and carotid-to-descending bypass (95). While these approaches may be adequate in adults, this strategy is not optimal for younger patient for whom there is the need to account for somatic growth.
A major challenge for patients with aortic anomalies resulting in tracheobronchial compression is that the true denominator of patients with each anomaly, i.e., circumflex aorta, hairpin aorta, or midline descending thoracic aorta, is not known. In patients with severe airway and esophageal symptoms or with recurrent symptoms following prior vascular ring repair, we have taken an aggressive approach of offering aortic reconstruction. For first-time patients with primarily swallowing difficulty, our approach has been less aggressive.
Aneurysms causing central airway compression
Aneurysmal dilatation of either the aorta or pulmonary artery may lead to central airway compression. In older patients with aortic arch aneurysms causing bronchial compression, total arch replacement is the standard practice. Pulmonary artery aneurysms may occur in patients with tetralogy of Fallot with absent pulmonary valve (96-99), patients with chronic pulmonary overcirculation or hypertension (100), or in connective tissue disorders (101). While techniques of pulmonary artery reduction plasty with Lecompte maneuver have been described for tetralogy of Fallot with absent pulmonary valve (98,102), our approach has been to resect a short segment of ascending aorta, perform a Lecompte maneuver, and completely replace both branch pulmonary arteries with dilatable exGraft (PECALabs, Pittsburgh, PA, USA) (103). Given the abnormal nature of the pulmonary arteries, there is the potential for subsequent aneurysmal degeneration if this tissue is left in place (104). Techniques for addressing the airway in these patients include anterior tracheopexy and tracheobronchial splinting.
Reoperation on vascular rings
Freedom from reoperation following vascular ring repair has been reported to be 86% to 90% at 10 to 20 years (5,23,105), though these reoperation rates may be underestimated as 74% (20/27) of reoperative vascular ring patients undergoing surgery at Boston Children’s Hospital were referred from outside facilities. The principle mechanisms of failure can be broadly categorized into ongoing vascular compression, development of constricting scar tissue reforming the vascular ring, tracheobronchomalacia (32,33), or a combination. Axial imaging with 3-dimensional reconstruction, 3-phase dynamic bronchoscopy, and esophagram are essential elements to the preoperative evaluation of these patients.
Ongoing vascular compression may be related to an aberrant subclavian artery if the prior operation was merely division of the ligamentum arteriosum to relieve the vascular ring. For these patients, reoperation with subclavian-to-carotid transposition is standard. Among patients for whom the subclavian was transposed to the carotid, failure to resect the subclavian flush with the aorta may result in a residual diverticulum of Kommerell. For these patients, mobilization of the aorta to re-resect the diverticulum of Kommerell is necessary. Failure to recognize a circumflex aorta or midline descending thoracic aorta at the initial operation may result in residual symptoms, and aortic reconstruction may be necessary.
Development of scar tissue where previously a ligamentum arteriosum or accessory aortic arch has been divided may result in reformation of the previously repaired vascular ring, and this often is apparent on esophagram. At the time of reoperation, dividing these adhesions and the accompanying fibrous bands on the esophagus along with more aggressive mobilization of the esophagus to allow it to rotate into the chest is our typical approach.
Finally, the infrequent application of tracheobronchopexy at the time of vascular ring operation appears to be a distinct mechanism of failure. Historically, patients with known tracheomalacia have been expected to “grow out of it”. In order to avoid these reoperations, our approach at the initial operation has evolved to include liberal use of tracheobronchopexy under bronchoscopic guidance for patients with tracheobronchomalacia and significant respiratory symptoms. Preoperative and intraoperative bronchoscopy is essential to distinguish between static airway compression from the vascular ring that may improve with vascular ring release and the presence of dynamic airway collapse or tracheobronchomalacia that may require additional tracheobronchopexy. Our goal is to achieve an almost normal appearing airway at the end of the operation. In order to achieve this, we will often employ the Munoz maneuver (negative suction test) intraoperatively in order to assess the effect of dynamic collapse following tracheobronchopexy. The Munoz maneuver involves applying up to 50 cmH2O of suction to the endotracheal tube while disconnected from the ventilator and observing for airway collapse bronchoscopically. In patients for whom there is greater than 50% collapse, additional airway work is required.
Bronchial compression following cardiac surgery
Airway compression arising as a complication of cardiac surgery has been reported following aortic arch reconstruction, arterial switch operation, and pulmonary artery stenting (75,106-115). Following aortic arch reconstruction, factors contributing to left main stem bronchial compression may include use of an overly large patch or direct implantation of the descending aorta on the distal ascending aorta without use of a patch. In the arterial switch operation, with or without arch reconstruction, the Lecompte maneuver necessarily forces the aorta to perform a hairpin turn from the ascending to the descending thoracic aorta. This may cause the left main stem bronchus to be squeezed between the ascending and descending thoracic aorta especially if there is subsequent aneurysmal dilatation of the neo-aortic root. Finally, in patients for whom the pulmonary arteries require stent placement due to stenosis or hypoplasia, the airway may become compressed between the stented pulmonary artery and the aorta. This has been reported in patients with truncus interrupted aortic arch (112,114), following Rastelli procedure (115), following unifocalization for tetralogy of Fallot with pulmonary atresia and major aortopulmonary collaterals (98), and single ventricle palliation requiring left pulmonary artery stenting (113). In all of these cases, patients will typically develop recurrent upper respiratory tract infections, wheezing, stridor, or dyspnea on exertion.
Despite the difference in mechanism when compared to patients with vascular rings or congenital airway compression syndromes, the diagnostic workup typically does not differ. Patients should undergo 3-phase bronchoscopy in order to determine the location, severity, and dynamic vs. static components of the compression. Patients should further undergo computed tomographic angiography in order to assess the vascular anatomy and correlate the findings with the bronchoscopy. The issue in these cases is usually that the space underneath the arch is inadequate for both the branch pulmonary artery and the mainstem bronchus. Aortopexy is not adequate given that posterior distraction of the aorta fails to increase the space underneath the arch.
In patients with an aneurysmal aortopulmonary amalgamation following single ventricle palliation, truncal root, or neo-aortic root following arterial switch operation, aortic root reduction is performed either with valve sparing aortic root replacement or aortic root and ascending aortoplasty (116-119). This in combination with either ascending aortic elongation or elongation of the proximal arch may substantially increase the space underneath the aortic arch relieving the compressive phenomenon (120).
A subset of older patients may benefit from descending aortic elongation using either Dacron or exGraft in order to increase the amount of space underneath the aortic arch (111). We tend not to favor descending aortic elongation in infants and young children, because implanting a graft of fixed size does not allow for subsequent growth potential. A theoretical benefit of the exGraft is the subsequent opportunity to dilate the graft in the catheterization laboratory.
For patients who have undergone pulmonary artery stenting with subsequent compression of the bronchus, reconstruction of the pulmonary artery with removal of most if not all of the stent is often helpful. The addition of a Lecompte maneuver may also be of benefit to free up space underneath the arch. Alternatively, in patients who have undergone single ventricle palliation, rerouting the left pulmonary artery to the innominate vein is a technique that has been described to achieve the same effect (121).
Management of the airway
Despite relief from compressing vessels, between 15% and 80% of patients with tracheobronchomalacia and tracheobronchial compression may continue to experience persistent postoperative residual respiratory symptoms of varying degrees (3,122). This may be related to the overall tendency not to perform concomitant tracheal surgery at the time of vascular ring division leaving the underlying tracheobronchomalacia unrepaired (3,21,23,45,105,122). Drawing from the esophageal atresia experience in which tracheomalacia is frequent (123-125), our group has liberally incorporated tracheobronchopexy into our management of patients with vascular rings and airway compression syndromes.
Tracheobronchomalacia is an innate weakness of the cartilaginous rings. This leads to widening of the posterior membranous trachea. With exhalation, either the cartilaginous aspect of the trachea or bronchus collapses or the membranous trachea intrudes posteriorly (Figure 8). Even with relief of the vascular compression, the abnormalities of the trachea remain.
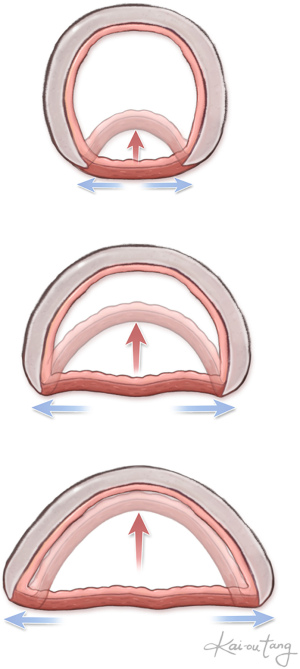
Tracheobronchopexy
The goal of posterior tracheobronchopexy is to fix the membranous trachea in place. After mobilizing the esophagus, non-absorbable monofilament sutures are placed as partial thickness bites into the membranous trachea. Depending on the proximity of the membranous trachea to the spine, a strut consisting of bovine pericardium or autologous pericardium may be necessary in order to avoid excessive tension on the membranous trachea (126,127). These sutures are tied under bronchoscopic guidance in order to ensure appropriate placement and tension.
An alternative to posterior tracheobronchopexy is anterior tracheobronchopexy (46). For anterior tracheopexy or mainstem bronchopexy, the strut is placed on the anterior surface of the airway. At the end of the case, the strut is brought through the sternal halves as they are reapproximated and then tensioned and secured under bronchoscopic guidance. For more distal struts on the mainstem bronchus, it is possible to raise a pectoralis flap in order to bring the strut lateral to the sternum to be secured to a rib. In either case, it is critical to avoid setting the tension of the strut until the end of the case with the sternum almost approximated; otherwise the geometry will change as the chest is closed.
Tracheobronchial splinting
Anterior and posterior tracheobronchopexy is most useful for patients with malacia resulting in anteroposterior collapse of the cartilages or excessive intrusion of the posterior membrane. However, a subset of patients will have either a “saber sheath” deformity, in which the anteroposterior dimension is greater than normal and there is lateral collapse (128,129), or circumferential tracheobronchomalacia, in which the there is a combination of both anteroposterior and lateral wall collapse (129,130). In these patients, neither anterior nor posterior tracheobronchopexy supports the airway adequately to prevent collapse. External splinting serves to address this by providing rigid support. Non-resorbable materials that have been used include Marlex mesh (131,132), a dental bar (133), and ringed polytetrafluoroethylene prosthesis (134). The use of non-resorbable materials introduces a theoretical risk for infection and erosion; additionally the non-resorbable prostheses do not allow for growth.
More recently, bioresorbable splints have been used to support the airway. Multiple groups have reported the use of 3-dimensional printing to create bioresorbable splints with good results (135-138). One challenge with 3-dimensional printing is the time-intensive production process. Our group prefers to use a bioresorbable plate, Rapidsorb (DePuy Synthes, West Chester, PA, USA), that easily deforms when heated in a hot water bath (79,111,139). This allows us to rapidly produce new splints and change the shape of the splints as necessary based on intraoperative bronchoscopy in order to achieve the optimal result.
Pulmonary artery sling and complete tracheal rings
Pulmonary artery slings occur when the left pulmonary artery arises from the right pulmonary artery and passes behind the trachea (Figure 9). Though not strictly a vascular ring or vascular-related aerodigestive compression syndrome, this lesion requires a similar diagnostic workup given the association with complete tracheal rings. Patients will have a transthoracic echocardiogram, computed tomographic angiography to assess both the vascular anatomy and the airway, and rigid bronchoscopy to confirm the presence and length of complete tracheal rings. Several techniques have been reported for reconstruction of the trachea including resection and reconstruction (140), pericardial tracheoplasty (141,142), tracheal allograft reconstruction (143-145), autologous tracheoplasty (146), and slide tracheoplasty (147-153). Our preference is to perform slide tracheoplasty on cardiopulmonary bypass with reimplantation of the left pulmonary artery. In order to reduce tension on the left pulmonary artery, we routinely create a flap of main pulmonary artery to form the back wall and reconstruct the anterior wall of the vessel with thin patch pulmonary homograft.
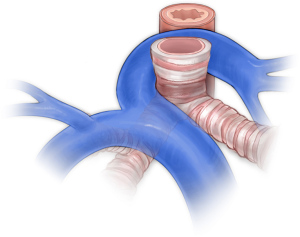
Complications
Chylothorax
The thoracic duct is highly variable and may consist of a single thoracic duct, two distinct trunks, or a plexus of lymphatics (154,155). Injury to the thoracic duct may result in high volume milky chest tube output after starting a diet. Pleural triglyceride >110 is highly suggestive of chylothorax (156,157).
Patients are typically placed on a low-fat diet with medium chain triglyceride supplementation and observed. Alternatively, patients who are breastfed may receive fortified skimmed breast milk (158). Failure of a low-fat diet necessitates a trial of nil per os with total parenteral nutrition. In the majority of cases, this is adequate (159,160). However, in a subset of patients who continue to experience large volume drainage, additional medical therapies may include treatment with corticosteroids (161), propranolol (162), or octreotide (163,164). Lymphangiography with thoracic duct embolization has successfully been employed (165,166), and mass ligation may be necessary in some cases (167). Upper extremity venous thrombosis, e.g., innominate or internal jugular vein, is a separate distinct cause for chylothorax. When this is the etiology of chylothorax, there is significant risk for failure of thoracic duct ligation (168).
Vocal cord paresis
In typical anatomy, the right recurrent laryngeal nerve branches off the vagus and wraps underneath the right subclavian artery before ascending in the tracheoesophageal groove to insert into the larynx. The left recurrent laryngeal nerve comes off the vagus nerve to pass underneath the aortic arch and posterior to the ductus arteriosus and then ascends the posterior aspect of the arch. In the setting of an aberrant right subclavian artery, patients will often have a non-recurrent right laryngeal nerve that has a direct course into the larynx. However, with an aberrant left subclavian artery, the left nerve will still wrap around the left sided ductal ligament. With double aortic arch, each nerve will wrap around the respective arch. Injury to the recurrent laryngeal nerve results in vocal cord palsy, which contributes to aspiration, clinically apparent dysphagia, and need for enteral feeding supplementation with a nasoenteric or gastrostomy tube (167,169).
During vascular ring repair, the risk for recurrent laryngeal nerve injury is approximately 4.5% to 8% (4,105,170), with a higher incidence—up to 19%—seen in reoperative vascular ring repair (33). Moreover, the incidence of vocal cord dysfunction has been reported as high as 48% to 59% in neonates after aortic arch reconstruction (171,172), and 20% to 25% in adults after total arch replacement (173,174). The incidence of vocal cord dysfunction appears to be directly related to whether screening is limited to symptomatic patients or if all patients are universally screened (167). Given that many seemingly asymptomatic patients may experience silent aspiration, it is our practice to routinely screen all patients before and after each operation. Our group also routinely uses intraoperative recurrent laryngeal nerve monitoring.
Adjunctive techniques
Intraoperative recurrent laryngeal nerve monitoring
Given the high incidence and potential associated morbidity, our programmatic approach has been to work with the otorhinolaryngologists to perform routine preoperative and postoperative vocal fold assessment. Additionally, intraoperative use of recurrent laryngeal nerve monitoring with either the Nerve Integrity Monitoring integrated electrode endotracheal tube, which is available in sizes 5–9, or a custom constructed Dragonfly electrode endotracheal tube, which is used for patients requiring an endotracheal tube smaller than size 5, is routine (175,176). This requires that patients not receive muscle relaxant until after the majority of the dissection has been completed. This technique is particularly useful in reoperative settings.
Esophageal mobilization
Between 43% and 50% of patients with vascular rings will have esophageal symptoms (3-5). Dissection of the fibrous bands to adequately mobilize the esophagus has always been an important part of relieving the dysphagia symptoms for patients with dysphagia lusoria (33,177,178). However, mobilization of the esophagus also serves to relieve direct vascular compression and also make space for posterior tracheopexy. We routinely perform these operations as a combined procedure with a pediatric general surgeon who specializes in pediatric esophageal disease (BZ).
A distinct challenge in the current management of this population of patients is determining an optimal assessment of esophageal function both pre- and post-operatively. While it is routine to perform esophagram in the preoperative assessment, subsequent evaluation of esophageal symptoms is not standardized. Whether this is to take the form of repeat esophagram, radionuclide motility study, or esophageal manometry is not certain and is an area ripe for investigation.
Artery of Adamkiewicz
The artery of Adamkiewicz has long been thought to be the primary blood supply to the anterior spinal cord between T8 and the conus medullaris. For this reason, either identification of the artery of Adamkiewicz with subsequent reimplantation or empiric reimplantation of intercostal vessels is routine at many centers when performing descending and thoracoabdominal aortic aneurysm repair (179-182). In the majority of cases, the artery of Adamkiewicz arises from between T8 and T12 (35,180,183), though up to 7.5% of patients will have the artery of Adamkiewicz identified at T7 or above (35). Additionally, in a minority (10–15%) of patients the artery of Adamkiewicz may not be identifiable (180,184). There is emerging evidence that the blood supply of the spinal cord may not be solely dependent on the artery of Adamkiewicz but on a vast network of spinal cord collateral vessels, the largest of which is the artery of Adamkiewicz (185-187). Despite the potential for some variance in vascular supply to the spinal cord, it is important to note that paraplegia is perhaps the most feared complication of any aortic operation. For this reason, our approach is to attempt to identify the artery of Adamkiewicz in patients who are undergoing complex aortic reconstruction or for whom significant aortopexy is expected.
Conclusions
Vascular rings and aerodigestive vascular-related compression syndrome are highly variable with vascular, airway, and esophageal elements contributing to an overall complex picture. A standardized approach to diagnostic evaluation allows for adequate operative planning. Moreover, with the broad spectrum of disease, the surgeon must have a variety of techniques available to address each distinct feature. Due to the complexity of these patients, a comprehensive approach requires coordination among congenital heart surgeons, pediatric surgeons, orthopedic surgeons, otorhinolaryngologists, plastic surgeons, pulmonologists, cardiologists, gastroenterologists, radiologists, and intensivists in addition to a dedicated team of nurses, advanced practice practitioners, respiratory therapists, speech and language pathologists, occupational therapists, and physical therapists, is needed.
Acknowledgments
Funding: None.
Footnote
Provenance and Peer Review: This article was commissioned by the Guest Editors (Antonio F. Corno and Jorge D. Salazar) for the column “Pediatric Heart” published in Translational Pediatrics. The article has undergone external peer review.
Conflicts of Interest: All authors have completed the ICMJE uniform disclosure form (available at https://tp.amegroups.com/article/view/10.21037/tp-23-39/coif). The column “Pediatric Heart” was commissioned by the editorial office without any funding or sponsorship. The authors have no other conflicts of interest to declare.
Ethical Statement: The authors are accountable for all aspects of the work in ensuring that questions related to the accuracy or integrity of any part of the work are appropriately investigated and resolved.
Open Access Statement: This is an Open Access article distributed in accordance with the Creative Commons Attribution-NonCommercial-NoDerivs 4.0 International License (CC BY-NC-ND 4.0), which permits the non-commercial replication and distribution of the article with the strict proviso that no changes or edits are made and the original work is properly cited (including links to both the formal publication through the relevant DOI and the license). See: https://creativecommons.org/licenses/by-nc-nd/4.0/.
References
- Mitchell SC, Korones SB, Berendes HW. Congenital heart disease in 56,109 births. Incidence and natural history. Circulation 1971;43:323-32. [Crossref] [PubMed]
- Bjornard K, Riehle-Colarusso T, Gilboa SM, et al. Patterns in the prevalence of congenital heart defects, metropolitan Atlanta, 1978 to 2005. Birth Defects Res A Clin Mol Teratol 2013;97:87-94. [Crossref] [PubMed]
- Humphrey C, Duncan K, Fletcher S. Decade of experience with vascular rings at a single institution. Pediatrics 2006;117:e903-8. [Crossref] [PubMed]
- Shah RK, Mora BN, Bacha E, et al. The presentation and management of vascular rings: an otolaryngology perspective. Int J Pediatr Otorhinolaryngol 2007;71:57-62. [Crossref] [PubMed]
- Herrin MA, Zurakowski D, Fynn-Thompson F, et al. Outcomes following thoracotomy or thoracoscopic vascular ring division in children and young adults. J Thorac Cardiovasc Surg 2017;154:607-15. [Crossref] [PubMed]
- Tallarita T, Rogers RT, Bower TC, et al. Characterization and surgical management of aberrant subclavian arteries. J Vasc Surg 2023;77:1006-15. [Crossref] [PubMed]
- Dong S, Alarhayem AQ, Meier G, et al. Contemporary management and natural history of aberrant right subclavian artery. J Vasc Surg 2022;75:1343-1348.e2. [Crossref] [PubMed]
- Bloom JP, Attia RQ, Sundt TM, et al. Outcomes of open and endovascular repair of Kommerell diverticulum. Eur J Cardiothorac Surg 2021;60:305-11. [Crossref] [PubMed]
- Greenberg SB. Dynamic pulmonary CT of children. AJR Am J Roentgenol 2012;199:435-40. [Crossref] [PubMed]
- Ullmann N, Secinaro A, Menchini L, et al. Dynamic expiratory CT: An effective non-invasive diagnostic exam for fragile children with suspected tracheo-bronchomalacia. Pediatr Pulmonol 2018;53:73-80. [Crossref] [PubMed]
- Lee EY, Litmanovich D, Boiselle PM. Multidetector CT evaluation of tracheobronchomalacia. Radiol Clin North Am 2009;47:261-9. [Crossref] [PubMed]
- Ngerncham M, Lee EY, Zurakowski D, et al. Tracheobronchomalacia in pediatric patients with esophageal atresia: comparison of diagnostic laryngoscopy/bronchoscopy and dynamic airway multidetector computed tomography. J Pediatr Surg 2015;50:402-7. [Crossref] [PubMed]
- Kamran A, Jennings RW. Tracheomalacia and Tracheobronchomalacia in Pediatrics: An Overview of Evaluation, Medical Management, and Surgical Treatment. Front Pediatr 2019;7:512. [Crossref] [PubMed]
- Choi S, Lawlor C, Rahbar R, et al. Diagnosis, Classification, and Management of Pediatric Tracheobronchomalacia: A Review. JAMA Otolaryngol Head Neck Surg 2019;145:265-75. [Crossref] [PubMed]
- Majid A, Sosa AF, Ernst A, et al. Pulmonary function and flow-volume loop patterns in patients with tracheobronchomalacia. Respir Care 2013;58:1521-6. [Crossref] [PubMed]
- Loring SH, O'donnell CR, Feller-Kopman DJ, et al. Central airway mechanics and flow limitation in acquired tracheobronchomalacia. Chest 2007;131:1118-24. [Crossref] [PubMed]
- Boonjindasup W, Marchant JM, McElrea MS, et al. Pulmonary function of children with tracheomalacia and associated clinical factors. Pediatr Pulmonol 2022;57:2437-44. [Crossref] [PubMed]
- Crenesse D, Berlioz M, Bourrier T, et al. Spirometry in children aged 3 to 5 years: reliability of forced expiratory maneuvers. Pediatr Pulmonol 2001;32:56-61. [Crossref] [PubMed]
- Kampschmidt JC, Brooks EG, Cherry DC, et al. Feasibility of spirometry testing in preschool children. Pediatr Pulmonol 2016;51:258-66. [Crossref] [PubMed]
- Aurora P, Stocks J, Oliver C, et al. Quality control for spirometry in preschool children with and without lung disease. Am J Respir Crit Care Med 2004;169:1152-9. [Crossref] [PubMed]
- Woods RK, Sharp RJ, Holcomb GW 3rd, et al. Vascular anomalies and tracheoesophageal compression: a single institution's 25-year experience. Ann Thorac Surg 2001;72:434-9. [Crossref] [PubMed]
- Yu D, Guo Z, You X, et al. Long-term outcomes in children undergoing vascular ring division: a multi-institution experience. Eur J Cardiothorac Surg 2022;61:605-13. [Crossref] [PubMed]
- Binsalamah ZM, Ibarra C, John R, et al. Contemporary Midterm Outcomes in Pediatric Patients Undergoing Vascular Ring Repair. Ann Thorac Surg 2020;109:566-72. [Crossref] [PubMed]
- Hastreiter AR, D'Cruz IA, Cantez T, et al. Right-sided aorta. I. Occurrence of right aortic arch in various types of congenital heart disease. II. Right aortic arch, right descending aorta, and associated anomalies. Br Heart J 1966;28:722-39. [Crossref] [PubMed]
- D’Cruz IA, Cantez T, Namin EP, et al. Part II: Right Aortic Arch, Right Descending Aorta, and Associated Anomalies. Br Heart J 1966;28:725-39.
- Achiron R, Rotstein Z, Heggesh J, et al. Anomalies of the fetal aortic arch: a novel sonographic approach to in-utero diagnosis. Ultrasound Obstet Gynecol 2002;20:553-7. [Crossref] [PubMed]
- D'Antonio F, Khalil A, Zidere V, et al. Fetuses with right aortic arch: a multicenter cohort study and meta-analysis. Ultrasound Obstet Gynecol 2016;47:423-32. [Crossref] [PubMed]
- Knight L, Edwards JE. Right aortic arch. Types and associated cardiac anomalies. Circulation 1974;50:1047-51. [Crossref] [PubMed]
- FELSON B. PALAYEW MJ. THE TWO TYPES OF RIGHT AORTIC ARCH. Radiology 1963;81:745-59. [Crossref] [PubMed]
- Sobh DM, Batouty NM, Abdelwahab RM, et al. Ductus arteriosus location in relation to aortic arch position, branching pattern, and viscero-atrial situs. Clin Radiol 2019;74:732.e1-8. [Crossref] [PubMed]
- Backer CL, Mavroudis C, Rigsby CK, et al. Trends in vascular ring surgery. J Thorac Cardiovasc Surg 2005;129:1339-47. [Crossref] [PubMed]
- Backer CL, Mongé MC, Russell HM, et al. Reoperation after vascular ring repair. Semin Thorac Cardiovasc Surg Pediatr Card Surg Annu 2014;17:48-55. [Crossref] [PubMed]
- Labuz DF, Kamran A, Jennings RW, et al. Reoperation to correct unsuccessful vascular ring and vascular decompression surgery. J Thorac Cardiovasc Surg 2022;164:199-207. [Crossref] [PubMed]
- Shieh HF, Smithers CJ, Hamilton TE, et al. Descending Aortopexy and Posterior Tracheopexy for Severe Tracheomalacia and Left Mainstem Bronchomalacia. Semin Thorac Cardiovasc Surg 2019;31:479-85. [Crossref] [PubMed]
- Svetanoff WJ, Zendejas B, Frain L, et al. When to consider a posterolateral descending aortopexy in addition to a posterior tracheopexy for the surgical treatment of symptomatic tracheobronchomalacia. J Pediatr Surg 2020;55:2682-9. [Crossref] [PubMed]
- Burke RP, Wernovsky G, van der Velde M, et al. Video-assisted thoracoscopic surgery for congenital heart disease. J Thorac Cardiovasc Surg 1995;109:499-507; discussion 508. [Crossref] [PubMed]
- Arakawa H, Tokuyama K, Mochizuki H, et al. An unusual case of vascular ring: the right aortic arch with mirror-image branching and aneurysm of the left ductus arteriosus. Pediatr Pulmonol 1990;9:41-3. [Crossref] [PubMed]
- Schlesinger AE, Mendeloff E, Sharkey AM, et al. MR of right aortic arch with mirror-image branching and a left ligamentum arteriosum: an unusual cause of a vascular ring. Pediatr Radiol 1995;25:455-7. [Crossref] [PubMed]
- Zachary CH, Myers JL, Eggli KD. Vascular ring due to right aortic arch with mirror-image branching and left ligamentum arteriosus: complete preoperative diagnosis by magnetic resonance imaging. Pediatr Cardiol 2001;22:71-3. [Crossref] [PubMed]
- Mauchley DC, Stiegler JM, Padilla LA, et al. Repair of Rare Vascular Ring: Circumflex Aortic Arch with Associated Hypoplasia and Coarctation. World J Pediatr Congenit Heart Surg 2020;11:235-7. [Crossref] [PubMed]
- Fawcett SL, Gomez AC, Hughes JA, et al. Anatomical variation in the position of the brachiocephalic trunk (innominate artery) with respect to the trachea: a computed tomography-based study and literature review of Innominate Artery Compression Syndrome. Clin Anat 2010;23:61-9. [PubMed]
- Strife JL, Baumel AS, Dunbar JS. Tracheal compression by the innominate artery in infancy and childhood. Radiology 1981;139:73-5. [Crossref] [PubMed]
- Mustard WT, Bayliss CE, Fearon B, et al. Tracheal compression by the innominate artery in children. Ann Thorac Surg 1969;8:312-9. [Crossref] [PubMed]
- Gross RE, Neuhauser EB. Compression of the trachea by an anomalous innominate artery; an operation for its relief. Am J Dis Child (1911) 1948;75:570-4. [Crossref] [PubMed]
- Wine TM, Colman KL, Mehta DK, et al. Aortopexy for innominate artery tracheal compression in children. Otolaryngol Head Neck Surg 2013;149:151-5. [Crossref] [PubMed]
- Lawlor C, Smithers CJ, Hamilton T, et al. Innovative management of severe tracheobronchomalacia using anterior and posterior tracheobronchopexy. Laryngoscope 2020;130:E65-74. [Crossref] [PubMed]
- Moës CA, Izukawa T, Trusler GA. Innominate artery compression of the Trachea. Arch Otolaryngol 1975;101:733-8. [Crossref] [PubMed]
- Hawkins JA, Bailey WW, Clark SM. Innominate artery compression of the trachea. Treatment by reimplantation of the innominate artery. J Thorac Cardiovasc Surg 1992;103:678-82. [Crossref] [PubMed]
- Grimmer JF, Herway S, Hawkins JA, et al. Long-term results of innominate artery reimplantation for tracheal compression. Arch Otolaryngol Head Neck Surg 2009;135:80-4. [Crossref] [PubMed]
- Grillo HC, Wright CD, Dartevelle PG, et al. Tracheal compression caused by straight back syndrome, chest wall deformity, and anterior spinal displacement: techniques for relief. Ann Thorac Surg 2005;80:2057-62. [Crossref] [PubMed]
- Tsugawa C, Ono Y, Nishijima E, et al. Transection of the innominate artery for tracheomalacia caused by persistent opisthotonus. Pediatr Surg Int 2004;20:55-7. [Crossref] [PubMed]
- Hasegawa T, Oshima Y, Hisamatsu C, et al. Innominate artery compression of the trachea in patients with neurological or neuromuscular disorders. Eur J Cardiothorac Surg 2014;45:305-11. [Crossref] [PubMed]
- Taniguchi Y, Matsubayashi Y, Kato S, et al. Tracheal stenosis due to cervicothoracic hyperlordosis in patients with cerebral palsy treated with posterior spinal fusion: a report of the first two cases. BMC Musculoskelet Disord 2021;22:217. [Crossref] [PubMed]
- Tomita H, Shimotakahara A, Shimojima N, et al. Inverse T-shaped sternotomy as novel thoracoplasty for severe chest deformation and tracheal stenosis. Surg Case Rep 2021;7:194. [Crossref] [PubMed]
- Fujimoto Y, Hirose K, Ota N, et al. Suprasternal approach for impending tracheo-innominate artery fistula. Gen Thorac Cardiovasc Surg 2010;58:480-3; discussion 483-4. [Crossref] [PubMed]
- Kurose M, Takano K, Mitsuzawa H, et al. Tracheo-innominate artery fistula with severe motor and intellectual disability: incidence and therapeutic management. Int J Pediatr Otorhinolaryngol 2014;78:1348-51. [Crossref] [PubMed]
- Bayford D. An account of a singular case of obstructed deglutition. In: Mem Med Soc Lond; 1794:275-86.
- Murthy K, Mattioli L, Diehl AM, et al. Vascular ring due to left aortic arch, right descending aorta, and right patent ductus arteriosus. J Pediatr Surg 1970;5:550-4. [Crossref] [PubMed]
- Berman W Jr, Yabek SM, Dillon T, et al. Vascular ring due to left aortic arch and right descending aorta. Circulation 1981;63:458-60. [Crossref] [PubMed]
- Chen FL, Vick GW, Ge S. Left cervical aortic arch with right ligamentum arteriosum forming a vascular ring. Tex Heart Inst J 2008;35:78-9. [PubMed]
- Nguyen HH, Sheybani E, Manning P, et al. Left aortic arch, right descending aorta, and right patent ductus arteriosus: precise depiction of a rare vascular ring with cardiac computed tomography. Circulation 2015;131:e404-5. [Crossref] [PubMed]
- Gross RE. Surgical Treatment for Dysphagia Lusoria. Ann Surg 1946;124:532-4. [Crossref]
- Geiss D, Williams WG, Lindsay WK, et al. Upper extremity gangrene: a complication of subclavian artery division. Ann Thorac Surg 1980;30:487-9. [Crossref] [PubMed]
- Todd PJ, Dangerfield PH, Hamilton DI, et al. Late effects on the left upper limb of subclavian flap aortoplasty. J Thorac Cardiovasc Surg 1983;85:678-81. [Crossref] [PubMed]
- Lodge FA, Lamberti JJ, Goodman AH, et al. Vascular consequences of subclavian artery transection for the treatment of congenital heart disease. J Thorac Cardiovasc Surg 1983;86:18-23. [Crossref] [PubMed]
- Wells WJ, Castro LJ. Arm ischemia after subclavian flap angioplasty: repair by carotid-subclavian bypass. Ann Thorac Surg 2000;69:1574-6. [Crossref] [PubMed]
- Diemont FF, Chemla ES, Julia PL, et al. Upper limb ischemia after subclavian flap aortoplasty: unusual long-term complication. Ann Thorac Surg 2000;69:1576-8. [Crossref] [PubMed]
- van Bogerijen GH, Patel HJ, Eliason JL, et al. Evolution in the Management of Aberrant Subclavian Arteries and Related Kommerell Diverticulum. Ann Thorac Surg 2015;100:47-53. [Crossref] [PubMed]
- Konstantinou N, Antonopoulos CN, Tzanis K, et al. Systematic Review and Meta-Analysis of Outcomes After Operative Treatment of Aberrant Subclavian Artery Pathologies and Suggested Reporting Items. Eur J Vasc Endovasc Surg 2022;63:759-67. [Crossref] [PubMed]
- Chiu P, Goldstone AB, Schaffer JM, et al. Endovascular Versus Open Repair of Intact Descending Thoracic Aortic Aneurysms. J Am Coll Cardiol 2019;73:643-51. [Crossref] [PubMed]
- Chiu P, Perry S, Bernstein D, et al. Hybrid management of dysphagia lusoria in a boy with Duchenne's muscular dystrophy. Cardiol Young 2022;32:1842-4. [Crossref] [PubMed]
- Stephens EH, Pochettino A. Vascular ring and sling repair - addressing the root of the problem. Cardiol Young 2022;32:1019-20. [Crossref] [PubMed]
- Donnelly LF, Bisset GS 3rd, McDermott B. Anomalous midline location of the descending aorta: a cause of compression of the carina and left mainstem bronchus in infants. AJR Am J Roentgenol 1995;164:705-7. [Crossref] [PubMed]
- McKenzie ED, Roeser ME, Thompson JL, et al. Descending Aortic Translocation for Relief of Distal Tracheal and Proximal Bronchial Compression. Ann Thorac Surg 2016;102:859-62. [Crossref] [PubMed]
- Robotin MC, Bruniaux J, Serraf A, et al. Unusual forms of tracheobronchial compression in infants with congenital heart disease. J Thorac Cardiovasc Surg 1996;112:415-23. [Crossref] [PubMed]
- Kim YM, Yoo SJ, Kim WH, et al. Bronchial compression by posteriorly displaced ascending aorta in patients with congenital heart disease. Ann Thorac Surg 2002;73:881-6. [Crossref] [PubMed]
- Grillo HC, Wright CD. Tracheal compression with "hairpin" right aortic arch: management by aortic division and aortopexy by right thoracotomy guided by intraoperative bronchoscopy. Ann Thorac Surg 2007;83:1152-7. [Crossref] [PubMed]
- Mongé MC, Hauck AL, Popescu AR, et al. Descending Aortic Translocation and Right Pulmonary Artery Reimplantation for Midline Descending Aorta and Crossed Pulmonary Arteries in an Infant. World J Pediatr Congenit Heart Surg 2019;10:111-5. [Crossref] [PubMed]
- Chiu P, Jennings RW, Friedman KG, et al. Bronchomalacia in Right Aortic Arch Treated With Descending Aortic Translocation and Airway Splint. Ann Thorac Surg 2022;113:e187-9. [Crossref] [PubMed]
- O'Byrne ML, Rome N, Santamaria RW, et al. Intra-procedural Bronchoscopy to Prevent Bronchial Compression During Pulmonary Artery Stent Angioplasty. Pediatr Cardiol 2016;37:433-41. [Crossref] [PubMed]
- Planché C, Lacour-Gayet F. Aortic uncrossing for compressive circumflex aorta. 3 cases. Presse Med 1984;13:1331-2. [PubMed]
- Bruniaux J, Villain E, Sidi D, et al. Severe tracheo-bronchial compression caused by a circumflex aorta. Apropos of 3 cases treated by aortic uncrossing. Arch Mal Coeur Vaiss 1984;77:557-63. [PubMed]
- Russell HM, Rastatter JC, Backer CL. The Aortic Uncrossing Procedure for Circumflex Aorta. Oper Tech Thorac Cardiovasc Surg 2013;18:15-31. [Crossref]
- Kamran A, Friedman KG, Jennings RW, et al. Aortic uncrossing and tracheobronchopexy corrects tracheal compression and tracheobronchomalacia associated with circumflex aortic arch. J Thorac Cardiovasc Surg 2020;160:796-804. [Crossref] [PubMed]
- Bleakney CA, Zafar F, Fraser CD Jr. Circumflex right aortic arch with associated hypoplasia and coarctation: repair by aortic arch advancement and end-to-side anastomosis. Ann Thorac Surg 2011;91:624-6. [Crossref] [PubMed]
- Subramaniam KG, Marimuthu K, Manohar K, et al. Anterior arch translocation for coarctation of circumflex aorta using median sternotomy without cardiopulmonary bypass. J Thorac Cardiovasc Surg 2011;141:1543-5. [Crossref] [PubMed]
- Bryant R 3rd, Wallen W, Rizwan R, et al. Modified Aortic Uncrossing Procedure: A Novel Approach for Norwood Palliation of Complex Univentricular Congenital Heart Disease With a Circumflex Aorta. World J Pediatr Congenit Heart Surg 2017;8:507-10. [Crossref] [PubMed]
- Alsoufi B, Dydynski P, Austin E, et al. Simultaneous Norwood and Aortic Uncrossing in Neonate With Aortic Atresia and Right Circumflex Aorta. Semin Thorac Cardiovasc Surg 2020;32:563-8. [Crossref] [PubMed]
- Said SM, Marey G, Jakubowski L, et al. Aortic uncrossing procedure: When the right becomes left. JTCVS Tech 2020;4:239-42. [Crossref] [PubMed]
- Konstantinov IE, Puga FJ. Surgical treatment of persistent esophageal compression by an unusual form of right aortic arch. Ann Thorac Surg 2001;72:2121-3. [Crossref] [PubMed]
- Gil-Jaurena JM, Murtra M, Gonçalves A, et al. Aortic coarctation, vascular ring, and right aortic arch with aberrant subclavian artery. Ann Thorac Surg 2002;73:1640-2. [Crossref] [PubMed]
- Ahluwalia GS, Rashid AG, Griselli M, et al. Hypoplastic circumflex retroesophageal right-sided cervical aortic arch with unusual vascular arrangement and severe coarctation. Ann Thorac Surg 2007;84:1014-6. [Crossref] [PubMed]
- Garg P, Bishnoi AK, Sharma P, et al. Ventral aorta repair for retroesophageal circumflex aortic arch in an adult. J Card Surg 2013;28:645-7. [Crossref] [PubMed]
- Hurtado CG, Nelson JS. Concurrent double aortic arch and circumflex aorta repair in a symptomatic child: a case report. J Cardiothorac Surg 2022;17:148. [Crossref] [PubMed]
- Hellenbrand WE, Kelley MJ, Talner NS, et al. Cervical aortic arch with retroesophageal aortic obstruction: report of a case with successful surgical intervention. Ann Thorac Surg 1978;26:86-92. [Crossref] [PubMed]
- Rabinovitch M, Grady S, David I, et al. Compression of intrapulmonary bronchi by abnormally branching pulmonary arteries associated with absent pulmonary valves. Am J Cardiol 1982;50:804-13. [Crossref] [PubMed]
- Watterson KG, Malm TK, Karl TR, et al. Absent pulmonary valve syndrome: operation in infants with airway obstruction. Ann Thorac Surg 1992;54:1116-9. [Crossref] [PubMed]
- Greene CL, Mainwaring RD, Sidell D, et al. Lecompte Procedure for Relief of Severe Airway Compression in Children With Congenital Heart Disease. World J Pediatr Congenit Heart Surg 2019;10:558-64. [Crossref] [PubMed]
- Siddeek H, Lunos S, Thomas AS, et al. Long Term Outcomes of Tetralogy of Fallot With Absent Pulmonary Valve (from the Pediatric Cardiac Care Consortium). Am J Cardiol 2021;158:118-23. [Crossref] [PubMed]
- Kreibich M, Siepe M, Kroll J, et al. Aneurysms of the pulmonary artery. Circulation 2015;131:310-6. [Crossref] [PubMed]
- Chiu P, Irons M, van de Rijn M, et al. Giant Pulmonary Artery Aneurysm in a Patient With Marfan Syndrome and Pulmonary Hypertension. Circulation 2016;133:1218-21. [Crossref] [PubMed]
- Nölke L, Azakie A, Anagnostopoulos PV, et al. The Lecompte maneuver for relief of airway compression in absent pulmonary valve syndrome. Ann Thorac Surg 2006;81:1802-7. [Crossref] [PubMed]
- Chow OS, Hoganson DM, Kaza AK, et al. Early Infant Symptomatic Patients With Tetralogy of Fallot With Absent Pulmonary Valve: Pulmonary Artery Management and Airway Stabilization. Ann Thorac Surg 2020;110:1644-50. [Crossref] [PubMed]
- McDonnell BE, Raff GW, Gaynor JW, et al. Outcome after repair of tetralogy of Fallot with absent pulmonary valve. Ann Thorac Surg 1999;67:1391-5; discussion 1395-6. [Crossref] [PubMed]
- Naimo PS, Fricke TA, Donald JS, et al. Long-term outcomes of complete vascular ring division in children: a 36-year experience from a single institution. Interact Cardiovasc Thorac Surg 2017;24:234-9. [PubMed]
- Mitchell MB, Campbell DN, Toews WH, et al. Autograft aortic arch extension and sleeve resection for bronchial compression after interrupted aortic arch repair. Ann Thorac Surg 2002;73:1969-71. [Crossref] [PubMed]
- Maddali MM, Kandachar PS, Al-Hanshi S, et al. Mechanical cause for acute left lung atelectasis after neonatal aortic arch repair with arterial switch operation: Conservative management. Ann Card Anaesth 2017;20:252-5. [Crossref] [PubMed]
- An HS, Choi EY, Kwon BS, et al. Airway compression in children with congenital heart disease evaluated using computed tomography. Ann Thorac Surg 2013;96:2192-7. [Crossref] [PubMed]
- Sakai T, Miki S, Ueda Y, et al. Left main bronchus compression after aortic reconstruction for interruption of aortic arch. Eur J Cardiothorac Surg 1995;9:667-9. [Crossref] [PubMed]
- Worsey J, Pham SM, Newman B, et al. Left main bronchus compression after arterial switch for transposition. Ann Thorac Surg 1994;57:1320-2. [Crossref] [PubMed]
- Chiu P, Jennings RW, Marx G, et al. Aortic elongation and bronchial splint for late bronchial complication after neonatal arch reconstruction. JTCVS Tech 2021;8:126-8. [Crossref] [PubMed]
- Varenbut J, Vanderlaan RD, Lam CZ, et al. Anterior Translocation of the Right Pulmonary Artery to Relieve Bronchial Compression in Truncus Arteriosus With Interrupted Aortic Arch. World J Pediatr Congenit Heart Surg 2022;13:111-3. [Crossref] [PubMed]
- Grohmann J, Stiller B, Neumann E, et al. Bronchial compression following pulmonary artery stenting in single ventricle lesions: how to prevent, and how to decompress. Clin Res Cardiol 2016;105:323-31. [Crossref] [PubMed]
- Takeuchi K, Srivastava A, Steed DR. Bronchial compression as adverse effect of right pulmonary artery stenting in a patient with truncus arteriosus communis and interrupted aortic arch. Ann Pediatr Cardiol 2019;12:66-8. [Crossref] [PubMed]
- Núñez M, Beleña J, Cabeza R, et al. Bronchial compression due to stent placement in pulmonary artery in a child with congenital heart disease. Paediatr Anaesth 2005;15:1137-9. [Crossref] [PubMed]
- McElhinney DB, Reddy VM, Pian MS, et al. Compression of the central airways by a dilated aorta in infants and children with congenital heart disease. Ann Thorac Surg 1999;67:1130-6. [Crossref] [PubMed]
- Ko Y, Nomura K, Murayama F, et al. Posterior aortic plication for tracheal compression by aortic dilatation in infants with congenital heart disease. Interact Cardiovasc Thorac Surg 2021;33:496-7. [Crossref] [PubMed]
- Tan CW, Marathe SP, Kwon MH, et al. Ascending Aortoplasty in Pediatric Patients Undergoing Aortic Valve Procedures. Ann Thorac Surg 2021;112:855-61. [Crossref] [PubMed]
- Lancaster TS, Romano JC, Si MS, et al. Aortic valve repair using geometric ring annuloplasty in pediatric and congenital heart disease patients. J Thorac Cardiovasc Surg 2022; [Crossref] [PubMed]
- Brancaccio G, Trezzi M, Secinaro A, et al. Ascending aortic extension to increase aortopulmonary space after comprehensive stage II palliation. Interact Cardiovasc Thorac Surg 2022;34:613-5. [Crossref] [PubMed]
- Kaneko Y, Hirata Y, Yagyu K, et al. Left innominate vein-pulmonary artery shunt with Glenn anastomosis in a Fontan candidate with central pulmonary artery stenosis. Pediatr Cardiol 2003;24:516-9. [Crossref] [PubMed]
- Callahan CP, Merritt TC, Canter MW, et al. Symptom persistence after vascular ring repair in children. J Pediatr Surg 2020;55:2317-21. [Crossref] [PubMed]
- Spitz L, Kiely E, Brereton RJ. Esophageal atresia: five year experience with 148 cases. J Pediatr Surg 1987;22:103-8. [Crossref] [PubMed]
- Slany E, Holzki J, Holschneider AM, et al. Tracheal instability in tracheo-esophageal abnormalities. Z Kinderchir 1990;45:78-85. [PubMed]
- Fraga JC, Jennings RW, Kim PC. Pediatric tracheomalacia. Semin Pediatr Surg 2016;25:156-64. [Crossref] [PubMed]
- Shieh HF, Smithers CJ, Hamilton TE, et al. Posterior tracheopexy for severe tracheomalacia. J Pediatr Surg 2017;52:951-5. [Crossref] [PubMed]
- Shieh HF, Smithers CJ, Hamilton TE, et al. Posterior Tracheopexy for Severe Tracheomalacia Associated with Esophageal Atresia (EA): Primary Treatment at the Time of Initial EA Repair versus Secondary Treatment. Front Surg 2017;4:80. [Crossref] [PubMed]
- Greene R. "Saber-sheath" trachea: relation to chronic obstructive pulmonary disease. AJR Am J Roentgenol 1978;130:441-5. [Crossref] [PubMed]
- Gamsu G, Webb WR. Computed tomography of the trachea: normal and abnormal. AJR Am J Roentgenol 1982;139:321-6. [Crossref] [PubMed]
- Murgu SD, Colt HG. Tracheobronchomalacia and excessive dynamic airway collapse. Respirology 2006;11:388-406. [Crossref] [PubMed]
- Filler RM, Buck JR, Bahoric A, et al. Treatment of segmental tracheomalacia and bronchomalacia by implantation of an airway splint. J Pediatr Surg 1982;17:597-603. [Crossref] [PubMed]
- Vinograd I, Filler RM, Bahoric A. Long-term functional results of prosthetic airway splinting in tracheomalacia and bronchomalacia. J Pediatr Surg 1987;22:38-41. [Crossref] [PubMed]
- Catarino PA, Saito S, Westaby S. Dental bar tracheopexy in a neonate. Ann Thorac Surg 2001;72:943-4. [Crossref] [PubMed]
- Hagl S, Jakob H, Sebening C, et al. External stabilization of long-segment tracheobronchomalacia guided by intraoperative bronchoscopy. Ann Thorac Surg 1997;64:1412-20; discussion 1421. [Crossref] [PubMed]
- Zopf DA, Hollister SJ, Nelson ME, et al. Bioresorbable airway splint created with a three-dimensional printer. N Engl J Med 2013;368:2043-5. [Crossref] [PubMed]
- Les AS, Ohye RG, Filbrun AG, et al. 3D-printed, externally-implanted, bioresorbable airway splints for severe tracheobronchomalacia. Laryngoscope 2019;129:1763-71. [Crossref] [PubMed]
- Tsai AY, Moroi MK, Les AS, et al. Tracheal agenesis: Esophageal airway support with a 3-dimensional-printed bioresorbable splint. JTCVS Tech 2021;10:563-8. [Crossref] [PubMed]
- Yu D, Peng W, Mo X, et al. Personalized 3D-Printed Bioresorbable Airway External Splint for Tracheomalacia Combined With Congenital Heart Disease. Front Bioeng Biotechnol 2022;10:859777. [Crossref] [PubMed]
- Kamran A, Smithers CJ, Baird CW, et al. Experience with bioresorbable splints for treatment of airway collapse in a pediatric population. JTCVS Tech 2021;8:160-9. [Crossref] [PubMed]
- Mattingly WT Jr, Belin RP, Todd EP. Surgical repair of congenital tracheal stenosis in an infant. J Thorac Cardiovasc Surg 1981;81:738-40. [Crossref] [PubMed]
- Idriss FS, DeLeon SY, Ilbawi MN, et al. Tracheoplasty with pericardial patch for extensive tracheal stenosis in infants and children. J Thorac Cardiovasc Surg 1984;88:527-36. [Crossref] [PubMed]
- Cosentino CM, Backer CL, Idriss FS, et al. Pericardial patch tracheoplasty for severe tracheal stenosis in children: intermediate results. J Pediatr Surg 1991;26:879-84; discussion 885. [Crossref] [PubMed]
- Jacobs JP, Elliott MJ, Haw MP, et al. Pediatric tracheal homograft reconstruction: a novel approach to complex tracheal stenoses in children. J Thorac Cardiovasc Surg 1996;112:1549-58; discussion 1559-60. [Crossref] [PubMed]
- Elliott MJ, Haw MP, Jacobs JP, et al. Tracheal reconstruction in children using cadaveric homograft trachea. Eur J Cardiothorac Surg 1996;10:707-12. [Crossref] [PubMed]
- Jacobs JP, Quintessenza JA, Andrews T, et al. Tracheal allograft reconstruction: the total North American and worldwide pediatric experiences. Ann Thorac Surg 1999;68:1043-51; discussion 1052. [Crossref] [PubMed]
- Backer CL, Holinger LD, Mavroudis C. Congenital Tracheal Stenosis: Tracheal Autograft Technique. Oper Tech Thorac Cardiovasc Surg 2007;12:178-83. [Crossref]
- Tsang V, Murday A, Gillbe C, et al. Slide tracheoplasty for congenital funnel-shaped tracheal stenosis. Ann Thorac Surg 1989;48:632-5. [Crossref] [PubMed]
- Manning PB. Slide Tracheoplasty for Congenital Tracheal Stenosis. Oper Tech Thorac Cardiovasc Surg 2007;12:184-93. [Crossref]
- Manning PB, Rutter MJ, Lisec A, et al. One slide fits all: the versatility of slide tracheoplasty with cardiopulmonary bypass support for airway reconstruction in children. J Thorac Cardiovasc Surg 2011;141:155-61. [Crossref] [PubMed]
- Stephens EH, Eltayeb O, Mongé MC, et al. Pediatric Tracheal Surgery: A 25-Year Review of Slide Tracheoplasty and Tracheal Resection. Ann Thorac Surg 2020;109:148-53. [Crossref] [PubMed]
- Grillo HC, Wright CD, Vlahakes GJ, et al. Management of congenital tracheal stenosis by means of slide tracheoplasty or resection and reconstruction, with long-term follow-up of growth after slide tracheoplasty. J Thorac Cardiovasc Surg 2002;123:145-52. [Crossref] [PubMed]
- Butler CR, Speggiorin S, Rijnberg FM, et al. Outcomes of slide tracheoplasty in 101 children: a 17-year single-center experience. J Thorac Cardiovasc Surg 2014;147:1783-9. [Crossref] [PubMed]
- DeMarcantonio MA, Hart CK, Yang CJ, et al. Slide tracheoplasty outcomes in children with congenital pulmonary malformations. Laryngoscope 2017;127:1283-7. [Crossref] [PubMed]
- Davis HK. A statistical study of the thoracic duct in man. Am J Anat 1915;17:211-44. [Crossref]
- Rosenberger A, Abrams HL. Radiology of the thoracic duct. Am J Roentgenol Radium Ther Nucl Med 1971;111:807-20. [Crossref] [PubMed]
- Maldonado F, Hawkins FJ, Daniels CE, et al. Pleural fluid characteristics of chylothorax. Mayo Clin Proc 2009;84:129-33. [Crossref] [PubMed]
- Samanidis G, Kourelis G, Bounta S, et al. Postoperative Chylothorax in Neonates and Infants after Congenital Heart Disease Surgery-Current Aspects in Diagnosis and Treatment. Nutrients 2022;14:1803. [Crossref] [PubMed]
- Neumann L, Springer T, Nieschke K, et al. ChyloBEST: Chylothorax in Infants and Nutrition with Low-Fat Breast Milk. Pediatr Cardiol 2020;41:108-13. [Crossref] [PubMed]
- Chan EH, Russell JL, Williams WG, et al. Postoperative chylothorax after cardiothoracic surgery in children. Ann Thorac Surg 2005;80:1864-70. [Crossref] [PubMed]
- Biewer ES, Zürn C, Arnold R, et al. Chylothorax after surgery on congenital heart disease in newborns and infants -risk factors and efficacy of MCT-diet. J Cardiothorac Surg 2010;5:127. [Crossref] [PubMed]
- Loomba RS, Wong J, Davis M, et al. Medical Interventions for Chylothorax and their Impacts on Need for Surgical Intervention and Admission Characteristics: A Multicenter, Retrospective Insight. Pediatr Cardiol 2021;42:543-53. [Crossref] [PubMed]
- Corda R, Chrisomalis-Dring S, Crook S, et al. Propranolol treatment for chylothorax after congenital cardiac surgery. J Thorac Cardiovasc Surg 2022;163:1630-1641.e2. [Crossref] [PubMed]
- Caverly L, Rausch CM, da Cruz E, et al. Octreotide treatment of chylothorax in pediatric patients following cardiothoracic surgery. Congenit Heart Dis 2010;5:573-8. [Crossref] [PubMed]
- Costa KM, Saxena AK. Surgical chylothorax in neonates: management and outcomes. World J Pediatr 2018;14:110-5. [Crossref] [PubMed]
- Savla JJ, Itkin M, Rossano JW, et al. Post-Operative Chylothorax in Patients With Congenital Heart Disease. J Am Coll Cardiol 2017;69:2410-22. [Crossref] [PubMed]
- Majdalany BS, Saad WA, Chick JFB, et al. Pediatric lymphangiography, thoracic duct embolization and thoracic duct disruption: a single-institution experience in 11 children with chylothorax. Pediatr Radiol 2018;48:235-40. [Crossref] [PubMed]
- Orzell S, Joseph R, Ongkasuwan J, et al. Outcomes of Vocal Fold Motion Impairment and Dysphagia after Pediatric Cardiothoracic Surgery: A Systematic Review. Otolaryngol Head Neck Surg 2019;161:754-63. [Crossref] [PubMed]
- Nath DS, Savla J, Khemani RG, et al. Thoracic duct ligation for persistent chylothorax after pediatric cardiothoracic surgery. Ann Thorac Surg 2009;88:246-51; discussion 251-2. [Crossref] [PubMed]
- Kenny L, McIntosh A, Jardine K, et al. Vocal cord dysfunction after pediatric cardiac surgery: A prospective implementation study. JTCVS Open 2022;11:398-411. [Crossref] [PubMed]
- Said SM, Marey G, Knutson S, et al. Outcomes of Surgical Repair of Vascular Rings and Slings in Children: A Word for the Asymptomatic. Semin Thorac Cardiovasc Surg 2021;33:492-500. [Crossref] [PubMed]
- Pourmoghadam KK, DeCampli WM, Ruzmetov M, et al. Recurrent Laryngeal Nerve Injury and Swallowing Dysfunction in Neonatal Aortic Arch Repair. Ann Thorac Surg 2017;104:1611-8. [Crossref] [PubMed]
- Averin K, Uzark K, Beekman RH 3rd, et al. Postoperative assessment of laryngopharyngeal dysfunction in neonates after Norwood operation. Ann Thorac Surg 2012;94:1257-61. [Crossref] [PubMed]
- Ohta N, Kuratani T, Hagihira S, et al. Vocal cord paralysis after aortic arch surgery: predictors and clinical outcome. J Vasc Surg 2006;43:721-8. [Crossref] [PubMed]
- Fullmer T, Wang DC, Price MD, et al. Incidence and Treatment Outcomes of Vocal Fold Movement Impairment After Total Arch Replacement. Laryngoscope 2019;129:699-703. [Crossref] [PubMed]
- Lawlor CM, Meisner J, Jennings RW, et al. Comparative Effectiveness of Recurrent Laryngeal Nerve Monitoring Techniques in Pediatric Surgery. Laryngoscope 2022;132:889-94. [Crossref] [PubMed]
- Lawlor CM, Zendejas B, Baird C, et al. Intraoperative Recurrent Laryngeal Nerve Monitoring During Pediatric Cardiac and Thoracic Surgery: A Mini Review. Front Pediatr 2020;8:587177. [Crossref] [PubMed]
- Gross RE. Arterial malformations which cause compression of the trachea or esophagus. Circulation 1955;11:124-34. [Crossref] [PubMed]
- Lincoln JC, Deverall PB, Stark J, et al. Vascular anomalies compressing the oesophagus and trachea. Thorax 1969;24:295-306. [Crossref] [PubMed]
- Fischbein MP, Miller DC. Long-term durability of open thoracic and thoracoabdominal aneurysm repair. Semin Vasc Surg 2009;22:74-80. [Crossref] [PubMed]
- Tanaka H, Ogino H, Minatoya K, et al. The impact of preoperative identification of the Adamkiewicz artery on descending and thoracoabdominal aortic repair. J Thorac Cardiovasc Surg 2016;151:122-8. [Crossref] [PubMed]
- Uehara K, Matsuda H, Inoue Y, et al. Simple Identification of the Adamkiewicz Artery in Extended Thoracoabdominal Aortic Aneurysm Repair. Ann Thorac Surg 2017;103:e567-9. [Crossref] [PubMed]
- Afifi RO, Sandhu HK, Zaidi ST, et al. Intercostal artery management in thoracoabdominal aortic surgery: To reattach or not to reattach? J Thorac Cardiovasc Surg 2018;155:1372-1378.e1. [Crossref] [PubMed]
- Takase K, Sawamura Y, Igarashi K, et al. Demonstration of the artery of Adamkiewicz at multi- detector row helical CT. Radiology 2002;223:39-45. [Crossref] [PubMed]
- Taterra D, Skinningsrud B, Pękala PA, et al. Artery of Adamkiewicz: a meta-analysis of anatomical characteristics. Neuroradiology 2019;61:869-80. [Crossref] [PubMed]
- Etz CD, Kari FA, Mueller CS, et al. The collateral network concept: remodeling of the arterial collateral network after experimental segmental artery sacrifice. J Thorac Cardiovasc Surg 2011;141:1029-36. [Crossref] [PubMed]
- Griepp EB, Di Luozzo G, Schray D, et al. The anatomy of the spinal cord collateral circulation. Ann Cardiothorac Surg 2012;1:350-7. [PubMed]
- Yoshioka K, Tanaka R, Takagi H, et al. Systematic evaluation of collateral pathways to the artery of Adamkiewicz using computed tomography. Eur J Cardiothorac Surg 2018;54:19-25. [Crossref] [PubMed]