Hemostatic complications and systemic heparinization in pediatric post-cardiotomy veno-arterial extracorporeal membrane oxygenation failed to wean from cardiopulmonary bypass
Introduction
About 0.5–6% of pediatric patients receive veno-arterial extracorporeal membrane oxygenation (VA-ECMO) after cardiac surgery for cardiac and respiratory support (1,2). According to Extracorporeal Life Support Organization (ELSO) registry data, 44% of more than 10,000 neonatal cardiac ECMO patients survived to hospital discharge, and more than 15,000 pediatric cardiac ECMOs have an in-hospital mortality of 46% (3). The mortality is higher (55%) in children supported with ECMO who fail to wean from cardiopulmonary bypass (CPB) after cardiac surgery (4). Hemostatic complications, including bleeding and thrombosis, are the most common morbidity during ECMO, both of which further increase the risk of mortality (5,6). Pediatric ECMOs that fail to wean from CPB experience dilution coagulopathy, shear stress-related blood disruption, and inflammation-coagulation-fibrinolysis system activation (7). Thus, they are at high risk of bleeding in the early stage of ECMO, with the incidence as high as 20–70% (6,8,9). This high bleeding tendency leads to a clinical dilemma in choosing anticoagulation regimens, as there is no unified recommendation for practices related to peri-CPB unfractionated heparin (UFH) in pediatric VA-ECMO up until now (10,11). While some centers have adopted a strategy of delayed systemic heparinization (12), conclusions regarding its safety and practicability cannot be drawn due to insufficient patient numbers.
The aim of this retrospective observational study, which took place at a Chinese ECMO center, was to assess whether bleeding or thrombosis differ in VA-ECMO patients who were directly transitioned from CPB compared to those who received post-cardiotomy VA-ECMO for other indications. The impacts of hemostatic complications in early or late stages on in-hospital patients, as well as the association between the timing of systemic heparinization and hemostatic complications, were assessed in VA-ECMO patients who failed to wean from CPB. We present the following article in accordance with the STROBE reporting checklist (available at https://tp.amegroups.com/article/view/10.21037/tp-22-104/rc).
Methods
Patients and definitions
First, data from 153 continuous pediatric patients (aged less than 18 years) who received ECMO from January 2005 to June 2020 at Fuwai Hospital were collected. All patients (n=96) from this data set who had received post-cardiotomy VA-ECMO support due to failure to wean from CPB were included in the analysis (CPB-ECMO group). For comparison, critically ill children (n=50) who required VA-ECMO support and were not directly transitioned from CPB after cardiac surgery were also assessed (non-CPB-ECMO group). Seven patients were excluded because they either: had an ECMO running time of less than 24 hours (4 cases) or had used an anticoagulant other than UFH (3 cases). According to in-hospital mortality, the patients in the CPB-ECMO group were divided into two groups, survivors (n=46) and non-survivors (n=50). This study was conducted in accordance with the Declaration of Helsinki (as revised in 2013) and was approved by the institutional ethics board of Fuwai Hospital (No. 2020-1346). Individual consent for this retrospective analysis was waived.
Hemostatic complications are classified as major bleeding, circuit change, hemolysis, and thrombocytopenia. Major bleeding included re-exploration due to surgical site or cannula site bleeding, gastrointestinal bleeding, pulmonary hemorrhage, and intracranial hemorrhage (13,14). Hemolysis was captured when plasma-free hemoglobin (pFHb) levels greater than 50 mg/dL were detected. Thrombocytopenia was classified as having median platelet (PLT) counts less than 50×109/L during ECMO. Early hemostatic complications were those that occurred during the first 48 hours after VA-ECMO initiation. Otherwise, they were considered late complications.
Data from all patients were evaluated according to two scientific scores. First, the Society of Thoracic Surgeons-European Association for Cardio-Thoracic Surgery (STAT) mortality category distinguishes the complexity of cardiac surgery and has a high predictive value for postoperative mortality (15). Second, the Pediatric Extracorporeal Membrane Oxygenation Prediction (PEP) model weighs the patient’s age, indications [extracorporeal cardiopulmonary resuscitation (ECPR), cardiac, respiratory], arterial blood hydrogen ion concentration (PH), partial thromboplastin time (PTT), international normalized ratio (INR), and documented blood infections at the time of ECMO implantation. It is used for risk stratification in children supported by ECMO and is an effective tool for benchmarking pediatric ECMO populations (16). It was calculated in this study as described by the Collaborative Pediatric Critical Care Research Network (CPCCRN) (https://www.cpccrn.org/calculators/ecmoprediction/).
ECMO system
VA-ECMO was indicated as being utilized under the following circumstances in our center: (I) failure to wean from CPB; (II) post-cardiotomy cardiac arrest (CA) resuscitation (ECPR); (III) low cardiac output syndrome (LCOS) with no response to maximal medical treatment; and (IV) acute respiratory distress syndrome (ARDS) due to post-cardiotomy lung injury or pulmonary infection (14).
The ECMO system consists of a centrifugal pump (Jostra; Maquet Inc., Rastatt, Germany), a membrane oxygenator (Hilite 800/2400LTTM, Medos Medizintechnik AG, Stolberg, Germany; Quadrox PLS® MAQUET Cardiovascular, Hirrlingen, Germany; Sorin, Italy), and polyvinyl chloride tubing. Each ECMO system at the center was primed with crystal solution Plasma-Lyte A (PLA, Baxter Healthcare, Deerfield, IL, USA). Additionally, 20% human albumin, red blood cells (RBC), fresh frozen plasma (FFP), and sodium bicarbonate were used according to the individual patient’s needs.
ECMO implantation and peri-CPB anticoagulation practice in the CPB-ECMO group
Each ECMO system was implanted following failed attempts to wean the patient off CPB in the CPB-ECMO group. After the CPB to ECMO transition, an appropriate dose of protamine [0.5–0.8 mg protamine: 100 units (U) of UFH] was given to each patient to reverse systemic heparinization and counteract the high risk of bleeding during the ECMO initiation period. Each had a target activated clotting time (ACT) of 140–180 seconds (s). Systemic heparinization was delayed appropriately according to lower coagulation parameters: an activated partial thromboplastin time (APTT) of 50–70 s and an ACT of 140–180 s. Blood product transfusion for supplementing blood components was based on clinician bedside judgment without a protocolized approach. Once the patient’s condition had stabilized and chest-tube drainage had significantly reduced to below 2 mL/kg/h, a small dose of UFH (2–10 U/kg/h) was administered and the concentration was gradually increased.
ECMO implantation and early-stage anticoagulation practice in the non-CPB-ECMO group
Each VA-ECMO circuit was primed with 500–1,000 units UFH. Patients in the non-CPB-ECMO group received UFH bolus of 50 to 100 U/kg before cannulation in order to obtain an ACT of above 180 s. These patients were continuously infused with UFH at small doses after the initiation of VA-ECMO.
Anticoagulation maintenance
Continuous UFH infusion dosage was determined and regulated by the chest-tube drainage rate, APTT, and ACT. The APTT and ACT targets were 60–80 and 160–200 s, respectively. Although ACT has lower accuracy at lower heparin doses, it was used for convenient and rapid point-of-care monitoring to compensate for the time lag of APTT. Monitoring of APTT and ACT was carried out every 3 hours, while hemoglobin concentration, PLT count, prothrombin time, and INR were measured once or twice per day. From 2017 onwards, the monitoring was supplemented a combination of UFH activity tests (anti-factor Xa levels and antithrombin III activity), D-Dimer, fibrinogen, fibrinogen degradation products, and other parameters. The anticoagulation monitoring protocols at our center, including the test items, target range, and blood sampling times, are being continuously improved. The current target parameters are listed in Table S1.
The transfusion threshold for the patients was not fixed. RBCs were infused to achieve a hemoglobin target of above 100g/L, PLTs were infused when PLT counts were less than 50×109/L, and FFP was given to supplement coagulation factors and antithrombin III >50%. Argatroban was administered for heparin-induced thrombocytopenia.
During ECMO, the entire circuit was examined hourly, and the pFHb level was monitored daily. The ECMO circuit was replaced if there was evidence of clots in the circuit, including excessive hemolysis (pFHb >100 mg/dL), increased oxygenator delta pressure, reduced oxygen or carbon dioxide transfer from the oxygenator, or consumptive coagulopathy that required repeated transfusion of PLT and FFP. Other detailed approaches to the management of pediatric post-cardiotomy VA-ECMO have been previously described in literature (14).
Outcomes
(I) To compare the prevalence of hemostatic complications in the CPB-ECMO group with the non-CPB-ECMO group. (II) To assess the impacts of hemostatic complications in different stages on clinical outcomes in the CPB-ECMO group. (III) To explore the association between the timing of systemic heparinization and hemostatic complications at different stages.
Statistical analyses
All data were analyzed and visualized using SPSS 22.0 (SPSS, Inc., Chicago, IL, USA) and GraphPad Prism 8.0 (GraphPad Software, Inc., San Diego, CA, USA). Continuous variables were represented by their median (interquartile range) [M (Q25, Q75)], then analyzed using Mann-Whitney U test. Categorical variables were expressed as a percentage of n (%), then analyzed using Fisher’s exact test or chi-square test. The impact of the hemostatic complications at different stages on in-hospital mortality was assessed using univariate logistic regression. Multivariate logistic regression analysis was conducted to explore the association between the timing of systemic heparinization and the different stages-hemostatic complications after adjusting for confounding variables including illness severity and patient demographics at the time of ECMO implantation (age, weight, STAT category and PEP model). The receiver operating characteristic (ROC) curve of the model was developed and analyzed to explore the predictive value of the timing of systemic heparinization for hemostatic complications. The area under the ROC curve (AUROC) was used to assess discrimination, while the cut-off points were calculated by obtaining the best Youden index (sensitivity + specificity −1). We then used violin plots to depict the distribution of the primary exposures of interest (UFH start hours, chest-tube drainage, blood products transfusion) by early/non-early bleeding groups and the probability density of the data. Correlation between both the RBC and PLT transfusion rates was assessed using the Spearman rank correlation, while we used linear regression to test for correlation among chest-tube drainage rate, transfusion of blood products, circuit change time, first ECMO system duration, peak pFHb, and UFH start time. All statistical tests were two-tailed with the significance set to P<0.05.
Results
Comparison of the CPB-ECMO group with the non-CPB-ECMO group
We identified 96 children requiring ECMO support that were unable to wean from CPB successfully. We included 50 other post-cardiotomy pediatric patients on ECMO for other indications as the control group. In-hospital mortality was comparable between the two groups (52.1% vs. 62.0%), and patient characteristics are further described in Table 1. Once ECMO was implanted, disease severity determined by the PEP model and STAT category were comparable in both groups, though patients in the CPB-ECMO group experienced much longer CPB compared to the control group. It should be noted that patients in the two groups were diagnosed with congenital heart disease (CHD) of high heterogeneity. The most common diagnosis was the transposition of great arteries (TGA) (19.9%) (Table S2), for which arterial switch operation (ASO) was the most common operation (25.3%) (Table S3).
Table 1
Variables | CPB-ECMO | Non-CPB-ECMO | |||||
---|---|---|---|---|---|---|---|
All (n=96) | Survivors (n=46) | Non-survivors (n=50) | Pa value | All (n=50) | Pb value | ||
Male | 61 (63.5) | 30 (65.2) | 31 (62.0) | 0.833 | 30 (60.0) | 0.721 | |
Age (months) | 12.4 (6.4, 41.2) | 14.6 (6.4, 43.3) | 10.1 (5.5, 38.5) | 0.441 | 12.9 (6.4, 35.4) | 0.795 | |
Weight (kg) | 8.5 (6.0, 12.9) | 9.6 (6.0, 13.8) | 8.3 (6.0, 11.8) | 0.320 | 8.3 (6.4, 11.8) | 0.856 | |
Height (cm) | 72.5 (63.3, 90.0) | 77.0 (64.0, 92.0) | 69.5 (63.0, 86.5) | 0.281 | 71.5 (64.0, 88.3) | 0.759 | |
Redo-cardiac surgery | 25 (26.0) | 13 (28.3) | 12 (24.0) | 0.650 | 11 (22.0) | 0.688 | |
Infection before ECMO | 9 (9.4) | 4 (8.7) | 5 (10.0) | 1.000 | 4 (8.0) | 1.000 | |
STAT category | 4.0 (2.0, 4.0) | 3.0 (2.0, 4.0) | 4.0 (2.0, 4.0) | 0.205 | 3.0 (2.0, 4.0) | 0.385 | |
CPB time (min) | 259.5 (154.0, 359.0) | 272.5 (161.5, 376.5) | 246.0 (141.8, 332.0) | 0.550 | 185.0 (106.8, 244.0) | 0.001 | |
Clamp time (min) | 119.5 (78.3, 154.8) | 118.0 (79.8, 157.0) | 125.0 (75.8, 153.8) | 0.968 | 92.0 (54.3, 135.0) | 0.016 | |
MAP (mmHg) | 46.5 (40.0, 58.0) | 46.5 (40.0, 59.3) | 47.0 (38.8, 56.0) | 0.555 | 52.5 (40.0, 60.0) | 0.186 | |
VIS | 26.5 (16.0, 45.0) | 22.0 (15.8, 43.5) | 27.5 (17.5, 47.3) | 0.282 | 27.0 (18.0, 48.5) | 0.374 | |
Lactate (mmol/L) | 7.9 (4.9, 11.2) | 6.7 (4.8, 9.1) | 8.9 (5.5, 14.1) | 0.019 | 9.2 (6.1, 14.6) | 0.061 | |
Left heart unloading | 69 (71.9) | 34 (73.9) | 35 (70.0) | 0.821 | 35 (70.0) | 0.849 | |
PEP model (%) | 45.0 (40.0, 56.0) | 41.5 (36.0, 49.8) | 50.5 (42.0, 59.5) | 0.002 | 53.5 (41.0, 62.3) | 0.140 |
Continuous data are presented as median (interquartile range) and categorical data as n (percent). a, survivors vs. non-survivors in CPB-ECMO group; b, complete CPB-ECMO group vs. non-CPB-ECMO group. CPB, cardiopulmonary bypass; ECMO, extracorporeal membrane oxygenation; STAT, Society of Thoracic Surgeons-European Association for Cardio-Thoracic Surgery; MAP, mean arterial pressure; VIS, vasoactive-inotropic score; PEP, Pediatric Extracorporeal Membrane Oxygenation Prediction.
Major bleeding was diagnosed in 69.8% of patients, and nearly a half (49.3%, 33/67) of these cases occurred within 48 hours of ECMO initiation in the CPB-ECMO group. The prevalence of major bleeding, including early bleeding, was significantly lower in the control group. Re-exploration due to surgical or cannulation site bleeding, the main manifestation of major bleeding, was more common in the CPB-ECMO group (Table 2, Table S4). Furthermore, multivariate logistic regression analysis showed that failure to wean from CPB correlated positively with bleeding during ECMO after adjusting for illness severity (Table 3).
Table 2
Variables | CPB-ECMO | Non-CPB-ECMO | |||||
---|---|---|---|---|---|---|---|
All (n=96) | Survivors (n=46) | Non-survivors (n=50) | Pa value | All (n=50) | Pb value | ||
Management | |||||||
UFH start (h) | 15.3 (8.8, 24.9) | 12.7 (7.0, 21.1) | 17.3 (9.2, 27.1) | 0.117 | 0.0 (0.0, 0.0) | <0.001 | |
Median flow rate (mL/min) | 645.0 (520.0, 900.0) | 680.0 (535.0, 800.0) | 600.0 (510.0, 940.0) | 0.894 | 600.0 (520.0, 875.0) | 0.737 | |
Median pump speed (rpm) | 2,715.0 (2,550.0, 2,852.5) | 2,692.0 (2,540.0, 2,840.0) | 2,750.0 (2,580.0, 2,878.0) | 0.405 | 2,760.0 (2,600.0, 2,840.0) | 0.443 | |
Chest-tube days (d) | 8.0 (5.0, 12.0) | 9.0 (5.0, 13.0) | 8.0 (5.0, 12.0) | 0.373 | 8.0 (4.5, 14.3) | 0.926 | |
Chest-tube drainage (mL/kg/d) | 15.5 (9.6, 25.7) | 19.1 (10.0, 26.1) | 14.1 (8.3, 26.6) | 0.644 | 15.1 (8.9, 23.3) | 0.408 | |
Transfusion | |||||||
RBC transfusion (mL/kg/d) | 26.2 (17.6, 37.3) | 23.6 (15.2, 34.7) | 28.5 (20.0, 39.1) | 0.078 | 26.5 (18.0, 33.2) | 0.658 | |
PLT transfusion (mL/kg/d) | 7.4 (2.3, 14.2) | 6.9 (2.1, 12.0) | 8.1 (2.6, 19.7) | 0.232 | 8.0 (2.1, 14.1) | 0.830 | |
FFP transfusion (mL/kg/d) | 9.7 (6.0, 17.1) | 8.0 (6.0, 16.3) | 11.0 (5.9, 18.5) | 0.294 | 8.1 (4.2, 12.1) | 0.197 | |
Hemostatic complications | |||||||
Major bleeding | 67 (69.8) | 28 (60.9) | 39 (78.0) | 0.079 | 24 (48.0) | 0.012 | |
Early bleeding | 33 (34.4) | 11 (23.9) | 22 (44.0) | 0.053 | 8 (16.0) | 0.021 | |
Late bleeding | 34 (35.4) | 17 (37.0) | 17 (34.0) | 0.832 | 16 (32.0) | 0.717 | |
Re-exploration | 60 (62.5) | 28 (60.9) | 32 (64.0) | 0.834 | 20 (40.0) | 0.014 | |
Gastrointestinal bleeding | 8 (8.3) | 0 (0) | 8 (16.0) | 0.006 | 6 (12.0) | 0.557 | |
Pulmonary bleeding | 8 (8.3) | 1 (2.2) | 7 (14.0) | 0.061 | 5 (10.0) | 0.765 | |
Intracranial hemorrhage | 1 (1.0) | 0 (0) | 1 (2.0) | 1.000 | 1 (2.0) | 1.000 | |
Circuit change | 16 (16.7) | 3 (6.5) | 13 (26.0) | 0.013 | 13 (26.0) | 0.195 | |
Early circuit change | 3 (3.1) | 2 (4.3) | 1 (2.0) | 0.606 | 3 (6.0) | 0.412 | |
Late circuit change | 13 (13.5) | 1 (2.2) | 12 (24.0) | 0.002 | 11 (22.0) | 0.240 | |
Change time (h) | 65.0 (51.0, 79.8) | 48.0 (32.0, 65.0) | 67.0 (52.5, 86.5) | 0.082 | 82.5 (52.8, 104.5) | 0.240 | |
1st ECMO duration (h) | 101.6 (78.3, 145.8) | 106.0 (71.8, 159.3) | 97.5 (88.8, 129.8) | 0.689 | 100.0 (77.5, 149.3) | 0.947 | |
Hemolysis | 47 (49.0) | 14 (30.4) | 33 (66.0) | 0.001 | 27 (54.0) | 0.604 | |
Early hemolysis | 19 (19.8) | 5 (10.9) | 14 (28.0) | 0.043 | 13 (26.0) | 0.406 | |
Late hemolysis | 28 (29.2) | 9 (19.6) | 19 (38.0) | 0.071 | 14 (28.0) | 1.000 | |
pFHb max | 40.0 (20.0, 70.0) | 30.0 (20.0, 50.0) | 70.0 (40.0, 110.0) | <0.001 | 50.0 (30.0, 92.5) | 0.453 | |
Thrombocytopenia | 28 (29.2) | 4 (8.7) | 24 (48.0) | <0.001 | 19 (38.0) | 0.351 | |
PLT median (×109/L) | 62.5 (47.5, 77.0) | 68.0 (59.0, 83.3) | 53.8 (73.8, 74.6) | 0.001 | 54.8 (45.3, 77.6) | 0.421 | |
PLT nadir (×109/L) | 32.0 (23.0, 45.0) | 36.5 (28.0, 51.3) | 27.5 (21.8, 40.3) | 0.014 | 28.0 (20.8, 40.0) | 0.163 | |
Outcomes | |||||||
ECMO duration (h) | 123.0 (91.5, 167.0) | 100.6 (89.8, 135.3) | 145.5 (99.8, 214.3) | 0.001 | 128.5 (90.0, 204.8) | 0.616 | |
Successfully weaned from ECMO | 67 (69.8) | 46 (100.0) | 21 (42.0) | <0.001 | 32 (64.0) | 0.576 | |
30-day mortality | 36 (37.5) | 0 (0.0) | 36 (72.0) | <0.001 | 24 (48.0) | 0.288 | |
Hospital stay (d) | 43.0 (23.5, 65.0) | 51.5 (39.8, 84.3) | 25.5 (14.0, 45.3) | <0.001 | 35.0 (22.0, 56.3) | 0.406 | |
ICU stay (d) | 28.0 (12.3, 48.0) | 33.5 (23.8, 56.0) | 15.5 (7.0, 38.5) | <0.001 | 28.0 (12.3, 39.0) | 0.760 | |
Ventilation time (h) | 511.5 (211.3, 855.5) | 562.0 (284.5, 965.8) | 331.5 (146.3, 841.3) | 0.012 | 442.5 (210.0, 766.8) | 0.740 |
Continuous data are presented as median (interquartile range) and categorical data as n (percent). a, survivors vs. non-survivors in CPB-ECMO group; b, complete CPB-ECMO group vs. non-CPB-ECMO group. CPB, cardiopulmonary bypass; ECMO, extracorporeal membrane oxygenation; UFH, unfractionated heparin; RBC, red blood cell; PLT, platelet; FFP, fresh frozen plasma; pFHb, peak free-plasma hemoglobin; ICU, intensive care unit.
Table 3
Hemostatic complications | CPB-ECMO in all patients (n=146) | The timing of systemic heparinization in CPB-ECMO group (n=96) |
|||||
---|---|---|---|---|---|---|---|
OR | 95% CI | P value | OR | 95% CI | P value | ||
Major bleeding | 2.613 | 1.268, 5.383 | 0.009 | 1.034 | 0.989, 1.081 | 0.140 | |
Early bleeding | 2.819 | 1.194, 6.656 | 0.018 | – | – | – | |
Late bleeding | 1.154 | 0.543, 2.451 | 0.709 | 0.964 | 0.926, 1.004 | 0.079 | |
Circuit change | 0.520 | 0.220, 1.225 | 0.135 | 1.026 | 0.980, 1.073 | 0.274 | |
Early circuit change | 1.831 | 0.348, 9.641 | 0.475 | 0.966 | 0.880, 1.061 | 0.473 | |
Late circuit change | 2.043 | 0.811, 5.146 | 0.130 | 0.980 | 0.932, 1.030 | 0.418 | |
Hemolysis | 0.759 | 0.357, 1.616 | 0.474 | 1.057 | 1.013, 1.103 | 0.011 | |
Early hemolysis | 0.762 | 0.330, 1.756 | 0.523 | 1.049 | 1.004, 1.097 | 0.034 | |
Late hemolysis | 0.919 | 0.400, 2.111 | 0.842 | 1.018 | 0.977, 1.061 | 0.386 | |
Thrombocytopenia | 0.642 | 0.297, 1.389 | 0.260 | 0.960 | 0.917, 1.005 | 0.079 |
CPB, cardiopulmonary bypass; ECMO, extracorporeal membrane oxygenation; OR, odds ratio; CI, confidence interval.
Patient characteristics and clinical outcomes in the CPB-ECMO group
Non-survivors tended to be more critically ill at the time of ECMO initiation, with higher lactate and PEP model results in the patients who were directly transitioned from CPB to ECMO (Table 1). The PEP model showed high discrimination for in-hospital mortality as a risk adjustment tool in the CPB-ECMO group, which revealed an AUROC of 0.679 [95% confidence interval (CI): 0.571–0.787]. We further explored the associations among the PEP model with hemostatic complications, blood product transfusion, and other clinical outcomes. Except for early hemolysis [AUROC =0.619 (95% CI: 0.507–0.732)], the PEP model did not show predictive values for bleeding, circuit change, and thrombocytopenia. A weak correlation existed between RBC transfusion (P=0.005, r=0.229), hospital length of stay (P=0.003, r=−0.245), intensive care unit (ICU) stay (P=0.007, r=−0.221), and PEP model.
Chest-tube bleeding and blood product transfusion were common. All patients received RBC transfusions, 77 patients (80.2%) received PLT, and 93 patients (96.9%) received FFP. Acquired thrombocytopenia was an expected severe complication during ECMO. As a result, the prevalence of major bleeding was as high as 85.7% and the survival rate was only 13.8% in patients with thrombocytopenia. Notably, thrombocytopenia increased in-hospital mortality by 9-fold after adjusting for PLT transfusion.
Based on their timing, we divide the complications into early and late stages. We paid extreme attention to early hemostatic complications, as CPB-induced coagulopathy and bleeding tendency was prevalent in this high-risk population. The incidence of early bleeding and early hemolysis was lower in the survivors (Table 2). Furthermore, the univariate logistic regression analysis demonstrated that early bleeding was associated with a 2-fold increased risk of in-hospital mortality and early hemolysis increased in-hospital by 3-fold. Meanwhile, late circuit change significantly increased the odds of in-hospital mortality (Table 4).
Table 4
Hemostatic complications | OR | 95% CI | P value |
---|---|---|---|
Major bleeding | 2.279 | 0.933, 5.570 | 0.071 |
Early bleeding | 2.500 | 1.039, 6.015 | 0.041 |
Late bleeding | 0.879 | 0.380, 2.030 | 0.762 |
Circuit change | 5.036 | 1.332, 19.043 | 0.017 |
Early circuit change | 2.227 | 0.195, 25.419 | 0.519 |
Late circuit change | 14.211 | 1.766, 114.340 | 0.013 |
Hemolysis | 4.437 | 1.881, 10.468 | 0.001 |
Early hemolysis | 3.189 | 1.046, 9.724 | 0.041 |
Late hemolysis | 2.520 | 0.999, 6.358 | 0.050 |
Thrombocytopenia | 8.997 | 2.682, 30.184 | <0.001* |
RBC transfusion (mL/kg/d) | 1.022 | 0.997, 1.048 | 0.087 |
PLT transfusion (mL/kg/d) | 1.037 | 0.995, 1.080 | 0.082 |
FFP transfusion (mL/kg/d) | 1.036 | 0.988, 1.087 | 0.139 |
Chest-tube drainage (mL/kg/d) | 1.000 | 0.969, 1.032 | 0.997 |
*, we adjusted for PLT transfusion volume in the logistic regression analysis examining the association between thrombocytopenia and in-hospital mortality. OR, odds ratio; CI, confidence interval; RBC, red blood cell; PLT, platelet; FFP, fresh frozen plasma.
Patients with early bleeding also received larger amounts of RBC [32.0 (24.6, 40.1) vs. 22.7 (14.1, 36.6) mL/kg/d, P=0.009], PLT [9.5 (4.0, 22.0) vs. 6.6 (1.3, 13.0) mL/kg/d, P=0.029], and FFP [12.0 (7.3, 19.4) vs. 7.8 (4.8, 14.9) mL/kg/d, P=0.017], as well as more chest-tube drainage [23.8 (13.4, 33.0) vs. 13.0 (8.5, 22.2) mL/kg/d, P=0.001]. Thus, they were required to wait longer before starting the continuous UFH infusion [19.8 (15.0, 28.7) vs. 11.6 (7.1, 19.3) h, P=0.002] (Figure 1).
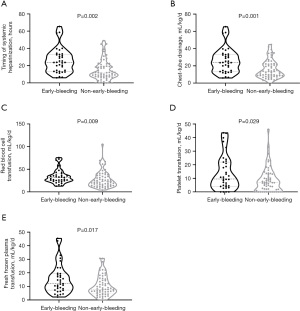
UFH-related anticoagulation practice and hemostatic complications
As shown in Table 2, the timing of systemic heparinization was 15.3 (8.8, 24.9) hours after ECMO implantation in the CPB-ECMO group. Patients received varying degrees of delayed systemic heparinization during the initial 48 hours to reduce early bleeding.
Spearman correlation analysis revealed significant relationships between peak pFHb, circuit change time, and UFH start time (Figure 2), while chest-tube drainage, transfusion of blood products, and first ECMO system duration were not apparently related to the timing of systemic heparinization.
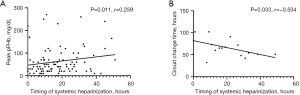
The timing of systemic heparinization correlated positively with hemolysis (Figure S1), especially early hemolysis, after adjusting for confounding factors in the multivariate regression analysis (Table 3). The UFH start hours had a predictive value for early hemolysis, and AUROC was 0.661 (95% CI: 0.531–0.791). The cut-off value of delayed systemic heparinization was 9.5 hours (sensitivity 79.7%, specificity 50.0%, P=0.031) (Figure 3). The incidence of early hemolysis below and above the cut-off point were 3.4% and 26.9% (P=0.010), respectively.
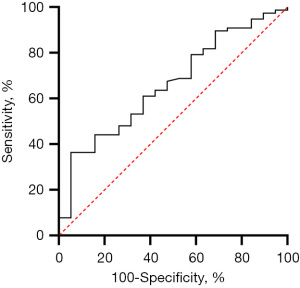
Discussion
The focus of this study was pediatric patients who had directly transitioned from CPB to VA-ECMO after cardiac surgery, and it included the non-CPB-ECMO group as a control. The major findings of our study are the following. (I) The risk of bleeding, especially early bleeding, was significantly increased in patients who failed to wean from CPB. (II) Early bleeding, early hemolysis, late circuit change, and thrombocytopenia significantly increased in-hospital mortality in the CPB-ECMO group. (III) Delayed systemic heparinization to avoid early bleeding increased early hemolysis in the CPB-ECMO group.
Pediatric post-cardiotomy patients on VA-ECMO who have directly transitioned from CPB experience a 3-fold increase in the incidence of early bleeding. Any bleeding is multifactorial (17). Post-cardiotomy dilutional coagulopathy, consumptive coagulopathy, acquired thrombocytopenia, and excessive anticoagulation can all lead to bleeding. The ELSO anticoagulation guideline (13) recommends that delayed systemic heparinization may be performed on patients who have had recent CPB or have existing bleeding. According to these guidelines, when a patient’s ACT drops to 300 s or below, the UFH infusion should be initiated with therapeutic anticoagulation of ACT at 180–220 s. However, an analysis of patients in the multisite bleeding and thrombosis on extracorporeal membrane oxygenation (BATE) study showed that ACT values between 160 and 180 s could reduce bleeding later, while a heparin dose greater than 40 IU/kg/hour prevented thrombosis later (18). There is no unified anticoagulation strategy for pediatric VA-ECMO patients after cardiac surgery, especially in the peri-CPB period (10,11). There has however been a general trend towards less aggressive anticoagulation strategies in adult ECMO. In fact, some centers have begun deferring systemic anticoagulation altogether in adult VA-ECMO (19), but there is no strong evidence that supports doing so in pediatrics as well.
Early bleeding accompanied with high levels of blood product transfusion increases the risk of mortality (20,21). To combat this, we accounted for chest tube drainage, APTT and ACT to adjust for bleeding and thrombosis. Most patients received delayed continuous UFH infusion to reduce early bleeding and blood products transfusion. Nellis et al. (22) reported that chest tube bleeding above 60 mL/kg/d was independently associated with worse clinical outcome. Muszynski et al. (23) showed that in the BATE study cohort, in which the RBC transfusion volume was 29.4 (17.4, 49.7) mL/kg/d and the PLT transfusion volume was 12.1 (6.8, 20.4) mL/kg/d on ECMO, the RBC transfusion volume independently increased the odds of mortality. Cashen et al. (24) reported that PLT transfusion volume was associated with an increased risk of mortality. Based on the above, we utilized the approach of delayed systemic heparinization and found that chest-tube drainage, RBC transfusion volume, and PLT transfusion volume in the CPB-ECMO group were lower than in their studies.
We found that the timing of systemic heparinization can predict early hemolysis, and the best Youden index is 9.5 hours. Hemorrhage and thrombosis are not completely independent processes; indeed, crossover exists between the two (25,26). Among the 67 patients with major bleeding, the incidence of circuit change was 19.4%, while hemolysis was 52.2%. These results are all higher than the average incidence for the CPB-ECMO group as a whole. Our results indicate that the timing of systemic heparinization has significant correlation with the peak pFHb and circuit change time. It can be said that hemolysis and thrombosis have a cause-and-effect relationship. Clots in the ECMO system cause blood damage, and an elevated pFHb has a predictive effect on thrombosis (6). This may be due to pFHb increasing von Willebrand factor (VWF)-mediated PLT adhesion in vitro under high shear stress, while also enhancing fibrin, extracellular matrix, and collagen-mediated microthrombus formation (27). It is worth noting that early hemolysis significantly increases in-hospital mortality (28). Additionally, pFHb can damage the kidney (29), as well as other target organs, which can lead to serious complications such as acute renal failure. The incidence of late circuit change in patients with early hemolysis was as high as 31.6%, and the two were significantly correlated (P=0.020).
In a previous study, von Stumm et al. (12) explored delayed systemic heparinization in 15 neonatal post-cardiotomy ECMOs for which the average UFH start time was 18.1±9.3 h. They found that delayed continuous UFH infusion could reduce bleeding and blood product transfusion without increased risk of thrombosis. This seems to be a bit different from our findings. Notably, our number of patients was much larger which thus increases the statistical significance; also, we analyzed the risk of hemolysis in addition to circuit change specifically. With this context, this study found that the timing of systemic heparinization cut-off value of 9.5 hours had a good Youden index to predict early hemolysis. This finding can be applied as a reference for planning anticoagulation management in the future.
The PEP model utilized in this study was developed using prospectively collected data from the BATE study (16) and was externally validated in the data from the ELSO registry (30). The score included the PH, PTT, and INR, which can indicate the severity of coagulopathy. Thus, it had a high degree of discrimination for in-hospital mortality in the CPB-ECMO group with multiple blood disorders at the time of ECMO initiation. Additionally, it was associated with RBC transfusion and early hemolysis. The PEP model is an effective tool in assisting clinicians in assessing and developing individualized anticoagulation regimens in pediatric post-cardiotomy VA-ECMO patients who failed to wean from CPB. Different congenital heart surgeries cause various degrees of PLT dysfunction and coagulopathy (31), so we adjusted for this confounding factor with the STAT category in multivariate logistic regression analysis.
In addition to accurate assessment of UFH timing, the coagulation factor levels, anticoagulant proteins, endothelial function, PLT function, and other factors are essential for a comprehensive evaluation of coagulation function (32,33). It is recommended to monitor the early pFHb more frequently to avoid early hemolysis. Given the heterogeneity of pediatric CHD patients, a thorough and specific anticoagulation monitoring protocol referring to the PEP model may benefit the clinical assessment of coagulopathy and the development of individualized anticoagulation strategies.
Strengths
This article compares pediatric post-cardiotomy VA-ECMO patients who failed to wean from CPB while using non-CPB-ECMO as a control to highlight the procedure’s high risk for bleeding. In addition, we discuss hemostatic complications by staging to further clarify the reasons and results of the delayed systemic heparinization in the CPB-ECMO group. These findings provided some basis for judging the situation of those special patients and implementing individualized anticoagulation strategies.
Limitations
This study did have some limitations. Firstly, this was a single-center retrospective study conducted at a single tertiary care medical center, a reduced context that ultimately restricts the generalizability of the findings. Secondly, we were unable to consider all confounding factors in the multivariate analysis, so residual confounding may have occurred. Finally, large scale multicenter prospective clinical trials are required before making meaningful changes to clinical practice in the future.
Conclusions
The direct transition from CPB to ECMO in pediatric post-cardiotomy patients significantly increases early bleeding. Delayed systemic heparinization to reduce early bleeding has good discrimination in predicting early hemolysis during ECMO. Coagulopathy is complex in pediatric post-cardiotomy VA-ECMO patients who failed to wean from CPB, and it is extremely important to monitor coagulation-related indicators in multiple dimensions to determine the timing of systemic heparinization.
Acknowledgments
The authors thank Mr. Ricardo Annon for his great contribution in language editing assistance.
Funding: This work was supported by CAMS Innovation Fund for Medical Sciences (CIFMS) (Nos. 2019XK320051, 2020-I2M-C&T-B-063).
Footnote
Reporting Checklist: The authors have completed the STROBE reporting checklist. Available at https://tp.amegroups.com/article/view/10.21037/tp-22-104/rc
Data Sharing Statement: Available at https://tp.amegroups.com/article/view/10.21037/tp-22-104/dss
Peer Review File: Available at https://tp.amegroups.com/article/view/10.21037/tp-22-104/prf
Conflicts of Interest: All authors have completed the ICMJE uniform disclosure form (available at https://tp.amegroups.com/article/view/10.21037/tp-22-104/coif). All authors report that this work was supported by CAMS Innovation Fund for Medical Sciences (CIFMS) (2019XK320051, 2020-I2M-C&T-B-063).
Ethical Statement: The authors are accountable for all aspects of the work in ensuring that questions related to the accuracy or integrity of any part of the work are appropriately investigated and resolved. The study was conducted in accordance with the Declaration of Helsinki (as revised in 2013). The study was approved by the institutional ethics board of Fuwai Hospital (No: 2020-1346) and individual consent for this retrospective analysis was waived.
Open Access Statement: This is an Open Access article distributed in accordance with the Creative Commons Attribution-NonCommercial-NoDerivs 4.0 International License (CC BY-NC-ND 4.0), which permits the non-commercial replication and distribution of the article with the strict proviso that no changes or edits are made and the original work is properly cited (including links to both the formal publication through the relevant DOI and the license). See: https://creativecommons.org/licenses/by-nc-nd/4.0/.
References
- Brunetti MA, Gaynor JW, Retzloff LB, et al. Characteristics, Risk Factors, and Outcomes of Extracorporeal Membrane Oxygenation Use in Pediatric Cardiac ICUs: A Report From the Pediatric Cardiac Critical Care Consortium Registry. Pediatr Crit Care Med 2018;19:544-52. [Crossref] [PubMed]
- Lorusso R, Raffa GM, Kowalewski M, et al. Structured review of post-cardiotomy extracorporeal membrane oxygenation: Part 2-pediatric patients. J Heart Lung Transplant 2019;38:1144-61. [Crossref] [PubMed]
- Extracorporeal Life Support Organization. ELSO Registry. Ann Arbor, MI; 2022. Available online: https://www.elso.org/Registry.aspx
- Sperotto F, Cogo P, Amigoni A, et al. Extracorporeal Membrane Oxygenation Support for Failure to Wean From Cardiopulmonary Bypass After Pediatric Cardiac Surgery: Analysis of Extracorporeal Life Support Organization Registry Data. Crit Care Explor 2020;2:e0183. [Crossref] [PubMed]
- Dalton HJ, Garcia-Filion P, Holubkov R, et al. Association of bleeding and thrombosis with outcome in extracorporeal life support. Pediatr Crit Care Med 2015;16:167-74. [Crossref] [PubMed]
- Dalton HJ, Reeder R, Garcia-Filion P, et al. Factors Associated with Bleeding and Thrombosis in Children Receiving Extracorporeal Membrane Oxygenation. Am J Respir Crit Care Med 2017;196:762-71. [Crossref] [PubMed]
- Fang ZA, Navaei AH, Hensch L, et al. Hemostatic Management of Extracorporeal Circuits Including Cardiopulmonary Bypass and Extracorporeal Membrane Oxygenation. Semin Thromb Hemost 2020;46:62-72. [Crossref] [PubMed]
- Barbaro RP, Paden ML, Guner YS, et al. Pediatric Extracorporeal Life Support Organization Registry International Report 2016. ASAIO J 2017;63:456-63. [Crossref] [PubMed]
- Basgoze S, Temur B, Aydın S, et al. Outcomes of Extracorporeal Membrane Oxygenation in Patients After Repair of Congenital Heart Defects. Pediatr Cardiol 2022; Epub ahead of print. [Crossref] [PubMed]
- Penk JS, Reddy S, Polito A, et al. Bleeding and Thrombosis With Pediatric Extracorporeal Life Support: A Roadmap for Management, Research, and the Future From the Pediatric Cardiac Intensive Care Society: Part 1. Pediatr Crit Care Med 2019;20:1027-33. [Crossref] [PubMed]
- Ozment CP, Scott BL, Bembea MM, et al. Anticoagulation and Transfusion Management During Neonatal and Pediatric Extracorporeal Membrane Oxygenation: A Survey of Medical Directors in the United States. Pediatr Crit Care Med 2021;22:530-41. [Crossref] [PubMed]
- von Stumm M, Subbotina I, Biermann D, et al. Impact of delayed systemic heparinization on postoperative bleeding and thromboembolism during post-cardiotomy extracorporeal membrane oxygenation in neonates. Perfusion 2020;35:626-32. [Crossref] [PubMed]
- Extracorporeal Life Support Organization. ELSO Anticoagulation Guideline 2014. Ann Arbor, MI; 2014. Available online: https://www.elso.org/Portals/0/Files/elsoanticoagulationguideline8-2014-table-contents.pdf
- Jin Y, Feng Z, Zhao J, et al. Outcomes and factors associated with early mortality in pediatric post-cardiotomy veno-arterial extracorporeal membrane oxygenation. Artif Organs 2021;45:6-14. [Crossref] [PubMed]
- Jacobs ML, O'Brien SM, Jacobs JP, et al. An empirically based tool for analyzing morbidity associated with operations for congenital heart disease. J Thorac Cardiovasc Surg 2013;145:1046-1057.e1. [Crossref] [PubMed]
- Bailly DK, Reeder RW, Winder M, et al. Development of the Pediatric Extracorporeal Membrane Oxygenation Prediction Model for Risk-Adjusting Mortality. Pediatr Crit Care Med 2019;20:426-34. [Crossref] [PubMed]
- Thomas J, Kostousov V, Teruya J. Bleeding and Thrombotic Complications in the Use of Extracorporeal Membrane Oxygenation. Semin Thromb Hemost 2018;44:20-9. [Crossref] [PubMed]
- Bailly DK, Reeder RW, Muszynski JA, et al. Anticoagulation practices associated with bleeding and thrombosis in pediatric extracorporeal membrane oxygenation; a multi-center secondary analysis. Perfusion 2022; Epub ahead of print. [Crossref] [PubMed]
- Wood KL, Ayers B, Gosev I, et al. Venoarterial-Extracorporeal Membrane Oxygenation Without Routine Systemic Anticoagulation Decreases Adverse Events. Ann Thorac Surg 2020;109:1458-66. [Crossref] [PubMed]
- Ellouze O, Abbad X, Constandache T, et al. Risk Factors of Bleeding in Patients Undergoing Venoarterial Extracorporeal Membrane Oxygenation. Ann Thorac Surg 2021;111:623-8. [Crossref] [PubMed]
- O'Halloran CP, Andren KG, Mecklosky J, et al. Mortality and Factors Associated With Hemorrhage During Pediatric Extracorporeal Membrane Oxygenation. Pediatr Crit Care Med 2020;21:75-81. [Crossref] [PubMed]
- Nellis ME, Dalton H, Karam O, et al. Quantifiable Bleeding in Children Supported by Extracorporeal Membrane Oxygenation and Outcome. Crit Care Med 2019;47:e886-92. [Crossref] [PubMed]
- Muszynski JA, Reeder RW, Hall MW, et al. RBC Transfusion Practice in Pediatric Extracorporeal Membrane Oxygenation Support. Crit Care Med 2018;46:e552-9. [Crossref] [PubMed]
- Cashen K, Dalton H, Reeder RW, et al. Platelet Transfusion Practice and Related Outcomes in Pediatric Extracorporeal Membrane Oxygenation. Pediatr Crit Care Med 2020;21:178-85. [Crossref] [PubMed]
- Giglia TM, Massicotte MP, Tweddell JS, et al. Prevention and treatment of thrombosis in pediatric and congenital heart disease: a scientific statement from the American Heart Association. Circulation 2013;128:2622-703. [Crossref] [PubMed]
- Vorisek CN, Sleeper LA, Piekarski B, et al. High-dose heparin is associated with higher bleeding and thrombosis rates in pediatric patients following cardiac surgery. J Thorac Cardiovasc Surg 2019;158:1199-206. [Crossref] [PubMed]
- Da Q, Teruya M, Guchhait P, et al. Free hemoglobin increases von Willebrand factor-mediated platelet adhesion in vitro: implications for circulatory devices. Blood 2015;126:2338-41. [Crossref] [PubMed]
- Lou S, MacLaren G, Best D, et al. Hemolysis in pediatric patients receiving centrifugal-pump extracorporeal membrane oxygenation: prevalence, risk factors, and outcomes. Crit Care Med 2014;42:1213-20. [Crossref] [PubMed]
- Lv L, Long C, Liu J, et al. Predictors of Acute Renal Failure During Extracorporeal Membrane Oxygenation in Pediatric Patients After Cardiac Surgery. Artif Organs 2016;40:E79-83. [Crossref] [PubMed]
- Bailly DK, Furlong-Dillard JM, Winder M, et al. External validation of the Pediatric Extracorporeal Membrane Oxygenation Prediction model for risk adjusting mortality. Perfusion 2021;36:407-14. [Crossref] [PubMed]
- Bailly DK, Boshkov LK, Zubair MM, et al. Congenital cardiac lesions involving systolic flow abnormalities are associated with platelet dysfunction in children. Ann Thorac Surg 2014;98:1419-24. [Crossref] [PubMed]
- Penk JS, Reddy S, Polito A, et al. Bleeding and Thrombosis With Pediatric Extracorporeal Life Support: A Roadmap for Management, Research, and the Future From the Pediatric Cardiac Intensive Care Society: Part 2. Pediatr Crit Care Med 2019;20:1034-9. [Crossref] [PubMed]
- Granja T, Hohenstein K, Schüssel P, et al. Multi-Modal Characterization of the Coagulopathy Associated With Extracorporeal Membrane Oxygenation. Crit Care Med 2020;48:e400-8. [Crossref] [PubMed]