3D-printed models optimize preoperative planning for pediatric cardiac tumor debulking
Introduction
Pediatric cardiac tumors are rare and benign approximately 90% of the time, but can cause clinically significant issues related to compression or infiltration of adjacent cardiopulmonary structures (1). This, in turn, can lead to pulmonary compromise, hemodynamic impairment, tachyarrhythmias or heart block, and even heart failure (1). Pediatric cardiac tumors have variable size and location in the heart, which often coincides with the type of symptoms displayed by the patient. When symptoms arise, tumor resection or partial debulking may be necessary to provide symptomatic relief and potentially a cure (2). These tumors are often first identified by echocardiography, but cardiac magnetic resonance (CMR) imaging is becoming the standard for imaging tissue characterization and classification. Nonetheless, biopsy remains the gold standard for definitive diagnosis (3). Computed tomography (CT) may be used in cases where hemodynamic compromise is a concern and rapid imaging is needed to identify tumor size and location to cardiac and thoracic structures. Though cross-sectional 2-dimensional (2D) imaging is useful for localizing tumors, it does not always capture the intricate interplay between invading tumor and critical local cardiac structures, such as the coronary arteries. In order to safely resect a cardiac tumor, it is crucial to understand the 3-dimensional (3D) relationship of the tumor to surrounding cardiac structures. Ultimately, this will improve operative approach and extent of resection. 3D-printed models have been used for tumor resection in adults (4); however, this study reports the first known application of 3D-printed heart models for preoperative planning of cardiac tumor debulking in pediatrics.
Methods
The institutional review board granted a waiver for this study. As such, informed consent was also waived to review these patients. Two pediatric patients were considered for this study. Patient 1 was a 2-month-old female, weighing 5.82 kg with a body surface area (BSA) of 0.32 m2. Patient 2 was a 12-day-old male weighing 2.94 kg with a BSA of 0.2 m2. Routine clinical procedures including echocardiography, CMR, and CT were performed for the two patients.
To create patient-specific 3D models of the heart and cardiac tumor, images from the CMR scan of patient 1 and CT of patient 2 were used. The 2D MR and CT images were used to generate a surface-rendered 3D reconstruction via Mimics Innovation Suite 3D visualization software interface (Materialise, Inc., Leuven, Belgium). Anatomical features of the heart and tumor were extracted from the 2D image stacks using image segmentation. Pixels with intensities that fell within the threshold range were selected (colored area in Figure 1) on the 2D images. The selected pixels belonging to each region of interest (e.g., the heart and tumor) were grouped. Following segmentation, the 2D images were reconstructed into a 3D stereolithographic (STL) volume representing the desired patient-specific heart-tumor model. Figure 2 shows the 3D STL models of the heart, represented by the cardiovascular blood volume, and cardiac tumor. The 3D heart-tumor STL file was then modified in 3Matic (Materialise Inc., Belgium) prior to sending for print. The cardiac tumor was made hollow while the heart was retained as a solid (Figure 3). PolyJet 3D printing technology was implemented using the Objet260 Connex3 (Stratasys, Ltd., Eden Prairie, MN, USA) printer in order to convert the STL file to physical models. The actual heart was 3D-printed by photopolymerization with opaque PolyJet resin (VeroWhite, Stratasys, Ltd., Eden Prairie, MN, USA), while the tumor was printed in a rubber-like transparent material (TangoPlus, Stratasys, Ltd., Eden Prairie, MN, USA). The material choice allowed for visualization of the cardiovascular structures within the tumor. The physical 3D-printed heart tumor models are shown in Figures 4 and 5. These models were used for preoperative planning of tumor debulking.
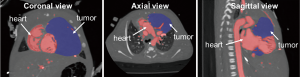
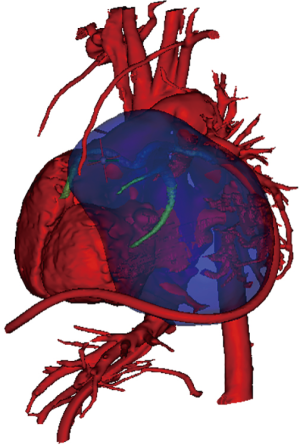
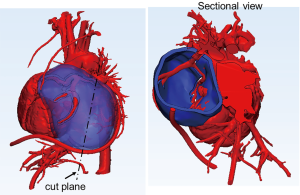
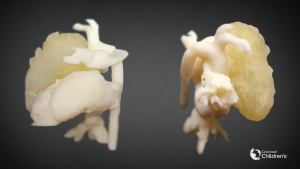
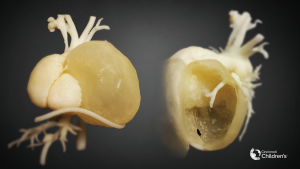
Results
Patient 1
Patient 1 was found to have cardiomegaly on chest X-ray along with oxygen saturations in the low 80% during evaluation in the emergency department for episodes of distress. Repeat echocardiography after drainage of a pericardial effusion revealed a large right ventricular (RV) tumor confirmed through CMR imaging (Figure 1). There was significant RV outflow tract (RVOT) obstruction with a 30-mmHg gradient and a patent foramen ovale leading to the decision to resect the tumor.
Analyzing the physical 3D heart-tumor model (Figure 4) prior to the operation, it was evident that the tumor involved the entire anterior surface of the heart, much of the main pulmonary artery, and covered the aortic root though they were not attached. Rightward the tumor extended to the right atrioventricular groove and overhung this area where the right coronary passed. The 3D model made it clearer that the tumor and right coronary could be separated. Much of the musculature of the RVOT and the free wall was replaced by tumor or was abnormally thin. The tumor wrapped around the main pulmonary artery and surrounded the left anterior descending (LAD) coronary artery as it emerged just lateral to the main pulmonary artery. Unlike viewing the CMR, the surgeon came to better understand the anatomical relationship and the extent to which the tumor could safely be resected without compromising the coronary arteries.
After establishing aorto-atrial cardiopulmonary bypass, the dissection began from the RV near the atrioventricular groove, taking extreme care to separate the right coronary artery from the tumor bulk. Frozen section revealed the tumor to have myxoma with fibroma characteristics. After consulting with oncology and cardiology, the decision was made to remove as much tumor as possible in an attempt to relieve RVOT obstruction. Venous drainage was converted to bicaval cannulation for optimal exposure, and the bulk of the tumor was progressively dissected from the RV, with particular attention to the mass compressing the RVOT. Once the tumor was debulked as far as was deemed safe, the majority of the anterior surface of the RV up to and into the mid pulmonary artery was excised since the pulmonary valve was involved. This large defect was reconstructed using CorMatrix (Aziyo Biologics, Inc., Richmond, CA, USA). The patient was transported to the cardiovascular intensive care unit (CVICU) in a stable condition and discharged 17 days later. Repeat CMR performed at 6 months postoperatively revealed that the remaining tumor was necrosing and regressing in size. At 2 years, there were no echocardiographic or CMR findings of left ventricular wall motion abnormality to suggest an ischemic insult or coronary perfusion disruption. The patient is thriving and will continue to be monitored.
Patient 2
Patient 2 was prenatally diagnosed with a large cardiac mass arising from the left ventricle (LV) and the left atrial wall, which was confirmed with CMR upon birth. The left coronary artery system traversed the mass, in particular, the left circumflex coronary artery which coursed over the superficial aspect of it. CMR tissue characterization included a differential diagnosis of fibroma versus rhabdomyoma, with more characteristic features of a fibroma. The mass appeared to be compressing the trachea so flexible bronchoscopy was performed which showed static and dynamic collapse of the trachea and the left main stem bronchus. Airway patency was possible with positive end-expiratory pressure to 15 cmH2O, which made long term tracheostomy not a good option. Additionally, with the airway compression concerns, it was discussed that a fibroma of this size would not completely regress and that a large rhabdomyoma would take an unknown amount of time to regress to the point that airway obstruction was relieved. Tumor debulking was planned to help relieve compression of the bronchus and allow for normalization in long-term pulmonary development. A 3D-printed model (Figure 5) was created to better understand the tumor location, size, coronary involvement, and proximity to the airway.
After median sternotomy, the heart was seen to be very malpositioned because of the tumor burden, rotating the heart counterclockwise with the right atrium posterior and the PA pushed rightward and anterior to the aorta. The patient had multiple arrhythmic episodes without any manipulation of the heart. After establishing aorto-bicaval cardiopulmonary bypass, the very apical portion of the tumor was removed and sent for frozen section. While waiting for this result, a conservative lateral and posterior resection of the tumor was completed to relieve airway compression, which was the primary reason to operate. Frozen section revealed rhabdomyoma, thus no further resection was warranted once airway compression was relieved as documented by bronchoscopy. The patient was weaned from bypass without signs of ischemia; however, there was severe mitral insufficiency previously ranging from mild to moderate throughout the fetal and pre-operative course. We attempted to improve the mitral valve function but it was not only difficult to visualize because the tumor bulk rotated the valve significantly but the entire posterior 2/3 portion of the annulus was tumor involving the posterior leaflet. Kaye annuloplasty stitches did little since the tumor bulk would not move. The anterior leaflet was adequate but did not coapt well to the rough tumor posterior surface, thus anterior leaflet extension was not pursued. Severe mitral regurgitation persisted, but since no tumor was resected in the area of the mitral valve annulus nor were there signs of ischemia of the papillary muscles, the hope was that this was temporary and that the valve would return to its pre-operative function with a few days of rest on extracorporeal membrane oxygenation (ECMO). The patient remained on ECMO and developed a lung infection, which did not allow weaning. After 2 weeks, the patient died of multi-organ failure.
Discussion
In adults, Al Jabbari et al. used 3D-printed models to better analyze two complex malignant cardiac tumors prior to resection (4). This technology has been translated into pediatrics to understand complex cardiac anatomy prior to many structural heart interventions. Various congenital heart anomalies have been printed for surgical planning including double-outlet right ventricle, atrial septal defects, tetralogy of Fallot and left heart hypoplasia (5). Valverde et al. used 3D modeling to plan the repair of transposition of the great arteries associated with a ventricular septal defect (VSD) and pulmonary stenosis (6). They reported that the relationship between the VSD, outlet septum and the great arteries was best identified using the 3D model. Kiraly et al. and Moore et al. reported the use of 3D printing for surgical planning of a complex aortic arch pathology (7,8). In both cases, the 3D model helped them to repair the obstruction and interruption, respectively, without using patch material by appreciating the exact locations of the pathology not demonstrable by other techniques.
The understanding of complex cardiac structures in 3D space remains a significant challenge in cardiology with current imaging techniques (9). 3D printing depicts anatomy in its native form, allowing for enhanced visualization and understanding of cardiac structures. We hope this will transplant into reduced cardiopulmonary bypass time and length of the operation. In the pediatric cardiac tumor cases, we report, the surgeon analyzed the spatial relationships for the planned resection and avoided any injury to critical structures while maximizing tumor resection. In both cases, the tumor involved the coronary arteries and was compressing nearby structures. The tumor vasculature and tissue margins could not be appreciated by traditional 2D imaging modalities. After careful planning with the 3D models, the tumors in both patients were successfully debulked while coronary blood flow was preserved, highlighting the utility of the 3D models in these cases. However, successful must be used cautiously since the second patient did not survive, but it is felt we did resect the maximal amount we could to relieve the airway obstruction without injuring the coronaries.
3D printing technology represents a paradigm shift in imaging that is enhancing surgical planning and education for healthcare providers and families. There are current limitations and challenges preventing 3D printing from being a widespread application. The image-based modeling including segmentation and reconstruction requires a significant amount of non-automated human input to produce the 3D models (9). Image segmentation and 3D reconstruction are skilled techniques that requires training and familiarizing with the software, leading to a potential source of error and possible bias (9). 3D reconstruction and printing are currently a time-consuming process that requires a dedication to details. At present, the technology is mostly available to teaching and research hospitals due to expense (10). It may become more clinically applicable and widespread through the development of a fully automated segmentation process and cheaper 3D printers (10). As the technology becomes more affordable and more widely available, 3D printing in pediatric cardiology could have an increasingly prominent role.
Furthermore, the method of 3D-printing patient-specific anatomies serves as a platform for other preoperative planning 3D applications such as augmented reality and virtual reality. For each of these applications, the same initial process of image acquisition, segmentation, and digital reconstruction is used to create a 3D rendering for virtual use. Augmented reality superimposes virtual objects onto real-time images while virtual reality creates a completely artificial computer simulated image and environment capable of real-time interaction (11). Both of these methods compliment 3D modeling with enhanced cardiac anatomy appreciation and allow surgeons to virtually manipulate objects or perform virtual surgeries. The authors are presently using these techniques to pre-operatively plan complex two ventricle repairs in heterotaxy patients. As the development of 3D technology progress, it has the potential to transform teaching methods and provide in-depth preoperative planning in the field of cardiothoracic surgery.
3D printing is also helpful for educational and training purposes as it provides physical models that help students and residents navigate complex pathological anatomy (9). 3D models can be used to engage patients and their families, providing them with a better understanding of their conditions. Building confidence in the surgical team and the proposed procedure remains one of the most critical parts of the surgeon-family relationship. In all, 3D printing is a growing field that has many integrative applications in the current practice of congenital cardiothoracic surgery.
Acknowledgements
The group would like to acknowledge Cincinnati Children’s MediaLab, led by director Ken Tegtmeyer, MD and lead animator Jeff Cimprich, for the production of Figures 2 and 5.
Footnote
Conflicts of Interest: Dr. DL Morales: CorMatrix Cardiovascular, Inc.—consultant, member of Medical Advisory Board, and recipient of research grants. The other authors have no conflicts of interest to declare.
Ethical Statement: The institutional review board granted a waiver for this study. As such, informed consent was also waived to review these patients.
References
- Tao TY, Yahyavi-Firouz-Abadi N, Singh GK, et al. Pediatric cardiac tumors: clinical and imaging features. Radiographics 2014;34:1031-46. [Crossref] [PubMed]
- Nield LE, Mendelson M, Ahmad N, et al. Clinical review of obstructive primary cardiac tumors in childhood. Congenit Heart Dis 2014;9:244-51. [Crossref] [PubMed]
- Beroukhim RS, Prakash A, Buechel ER, et al. Characterization of cardiac tumors in children by cardiovascular magnetic resonance imaging: a multicenter experience. J Am Coll Cardiol 2011;58:1044-54. [Crossref] [PubMed]
- Al Jabbari O, Abu Saleh WK, Patel AP, et al. Use of three-dimensional models to assist in the resection of malignant cardiac tumors. J Card Surg 2016;31:581-3. [Crossref] [PubMed]
- Vukicevic M, Mosadegh B, Min JK, et al. Cardiac 3D Printing and its Future Directions. JACC Cardiovasc Imaging 2017;10:171-84. [Crossref] [PubMed]
- Valverde I. Three-dimensional Printed Cardiac Models: Applications in the Field of Medical Education, Cardiovascular Surgery, and Structural Heart Interventions. Rev Esp Cardiol (Engl Ed) 2017;70:282-91. [Crossref] [PubMed]
- Kiraly L, Tofeig M, Jha NK, et al. Three-dimensional printed prototypes refine the anatomy of post-modified Norwood-1 complex aortic arch obstruction and allow presurgical simulation of the repair. Interact Cardiovasc Thorac Surg 2016;22:238-40. [Crossref] [PubMed]
- Moore RA, Wallen WJ, Riggs KW, et al. Three-dimensional printing in surgical planning: A case of aortopulmonary window with interrupted aortic arch. Ann Pediatr Cardiol 2018;11:201-3. [Crossref] [PubMed]
- Meier LM, Meineri M, Qua Hiansen J, et al. Structural and congenital heart disease interventions: the role of three-dimensional printing. Neth Heart J 2017;25:65-75. [Crossref] [PubMed]
- Byrne N, Velasco Forte M, Tandon A, et al. A systematic review of image segmentation methodology, used in the additive manufacture of patient-specific 3D printed models of the cardiovascular system. JRSM Cardiovasc Dis 2016;5. [Crossref] [PubMed]
- Khor WS, Baker B, Amin K, et al. Augmented and virtual reality in surgery-the digital surgical environment: applications, limitations and legal pitfalls. Ann Transl Med 2016;4:454. [Crossref] [PubMed]